ENABLING A NEW GENERATION OF SPACE TELESCOPES
Coherent has developed fabrication techniques which expand the use of metals and composites for spaceborne telescope mirrors.
February 8, 2024 by Coherent
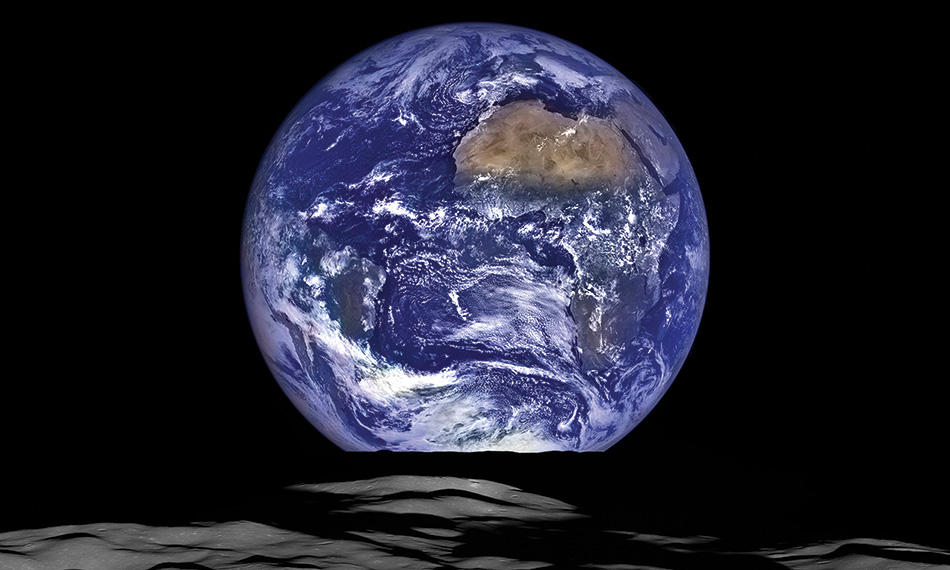
Today’s designers of space-based telescopes are borrowing a page from astronomy’s pioneers by returning to the use of metal mirrors. In 1668, Sir Isaac Newton used “speculum metal” to craft the mirror for the very first reflecting telescope ever made. Speculum is a copper-tin alloy that remained the telescope mirror material of choice until the mid-1800s. Then, technological advances caused a shift to glass mirrors which delivered better results.
Back to the Future with Metal Mirrors
So why turn the clock back to metal mirrors now? First because we’re not using speculum metal mirrors, which were hard to make and quickly tarnished. Today’s advanced spaceborne telescope mirrors are made from modern alloys of aluminum and beryllium, as well as composite materials, like silicon carbide (SiC).
A primary advantage of using these materials is that both the mirror and its mount (the mechanical structure that holds the mirror) can be produced as a single, monolithic structure. This isn’t practical with glass. Monolithic construction can dramatically reduce the part count and overall telescope weight, simplify and speed the assembly process, and, as a result, lower the cost.
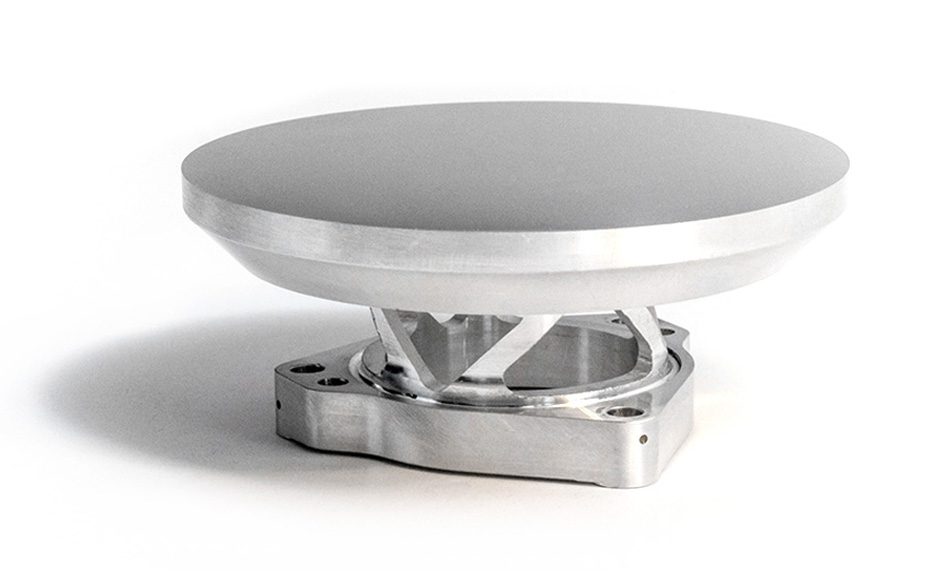
A monolithic metal mirror integrates the optical component with its mounting hardware.
Material Differences
While metal mirrors clearly offer substantial practical advantages, they’re not the “best” material for every application. This is especially true when considering the thermal characteristics of the telescope. This is a major consideration in spaceborne system design because the space environment exposes craft to temperature extremes and rapid temperature cycling. To explore this further, it’s useful to review some of the key properties of the materials most commonly used for spaceborne telescope primary mirrors.
Material |
Density (g/cm³) |
Stiffness (GPa) |
CTE (10⁶/°C) |
Thermal Conductivity (W/m·K) |
|
Glasses |
Fused silica |
2.2 |
73 |
0.55 |
1.4 |
ZERODUR® |
2.53 |
90 |
0.1 |
1.7 |
|
Composites |
SiC | 2.95 |
410 |
4 |
120 |
Metals |
Beryllium |
1.85 |
287 |
11.4 |
190 |
Aluminum |
2.7 |
69 |
23.6 |
205 |
ZERODUR® is a registered trademark of Schott AG
Fused silica and Zerodur are just two of several “glasses” used for mirror substrates, but they represent that class of materials well. Their defining characteristic is an extremely low coefficient of thermal expansion (CTE). This simply means these materials don’t expand or contract much with temperature changes.
Glasses also have low thermal conductivity. As a result, it takes a long time for them to either heat up or cool off in response to changes in ambient temperature. This keeps them stable when temperature changes.
In contrast, aluminum rapidly changes shape with temperature and also conducts heat well. It offers the practical advantages of being inexpensive and readily obtainable, and it can be quickly made into finished parts.
Beryllium is a bit of a “magical” material. It is less dense than glass but much stiffer. This makes it possible to fabricate thin, lightweight components from it that are still mechanically strong. Plus, at temperatures below about -130°C, beryllium changes crystalline form, and its thermal expansion coefficient becomes quite small. This combination of characteristics makes it the most lightweight, structurally strong, and thermally stable material available. That’s why it is used for the most demanding applications, like the primary mirror segments of the James Webb Space Telescope.
The negative of beryllium is that it’s rare and extremely expensive. Plus, it’s quite toxic so workers must take extreme precautions when processing it.
SiC has a higher density than any of these other materials. But that doesn’t mean that SiC mirrors can’t be made lightweight. This is because SiC is very stiff. As a result, SiC mirrors and structures can be made much thinner than with other materials, yet still remain quite strong and stable.
Together, these properties make glasses preferrable when the telescope is expected to experience large temperature gradients. This happens when one part of the telescope is in sunlight, which heats it up, while another part is in shadow. The shadowed part gets cold because its heat radiates away into space. The low CTE and thermal conductivity of glasses means the mirror won’t rapidly change shape in response to a temperature gradient.
In contrast, when temperatures are expected to be uniform across the structure, it’s advantageous to make both mirror and mount from a metal or composite. Their efficient heat dissipation enables such a system to reach equilibrium more quickly, reducing the impact of transient temperature changes.
Plus, when temperature does change, using the same material for both mirror and mount eliminates any difference in how they respond (even if they are separate pieces). This is important because differential expansion between the mirror and mount can throw a telescope out of focus. It can also put stress on the mirror and distort its surface shape which reduces image quality. Designers of glass mirror telescopes often create structures that minimize this effect, but this adds cost and sometimes weight.
Glass Has a Clear Advantage
In terms of optical fabrication, glass has long had one other important advantage over other materials. It can be shaped and polished to an extraordinarily high degree of precision. And it has been possible to make optics with this high precision for over a century–long before computers and lasers became available.
To understand exactly what “precision” means for telescope mirrors, it’s necessary to know a little bit about how optical surfaces are specified. There are essentially two separate domains of concern.
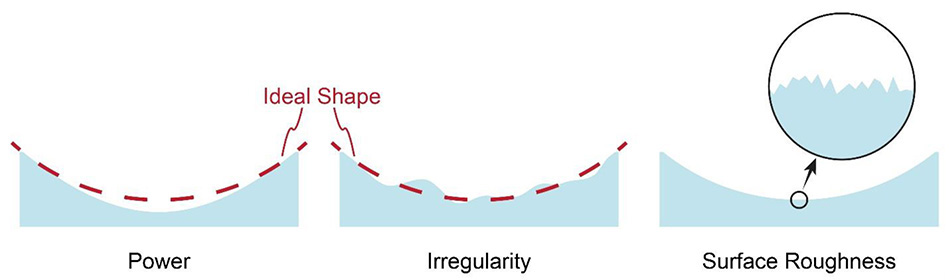
One way to quantify the shape of a telescope mirror is to measure its “power” (the amount by which its overall shape departs from the desired curve), its “irregularity” (the smaller scale bumps and ripples in the surface), and its “surface roughness” (the height variations on a microscopic scale).
The first is the overall shape, usually termed “figure” or “surface accuracy.” Specifically, this is how well the surface shape (curvature) of the mirror surface matches the design form.
For optimum image quality, the surface shape should match the intended value to within 150 nm or better (a nanometer is one billionth of a meter). It’s not at all unusual for the surface accuracy specification on a telescope mirror to be 60 nm.
The second area of concern is surface roughness on the microscopic scale. A telescope mirror must be extraordinarily smooth in order to minimize the amount of light it scatters. Scattered light lowers image brightness and contrast.
To form high-quality, visible wavelength images, a telescope mirror should have a maximum surface roughness of a few tens of Angstroms. An Angstrom is one ten-billionth of a meter. It’s about the diameter of a hydrogen atom.
In the past, it wasn’t possible to polish metal mirrors with either the shape accuracy or smoothness (low surface roughness) achievable with glass mirrors. To reduce metal mirror surface roughness, a thick layer (tens of microns) of metal was plated over the mirror to smooth it out.
For aluminum mirrors, it has been standard practice to use nickel as this plating material. But the CTE of nickel is different than aluminum. Differential expansion or contraction between the coating and mirror substrate can subsequently warp the mirror out of shape, reducing imaging quality. This has been a limiting factor in the deployment of aluminum mirrors.
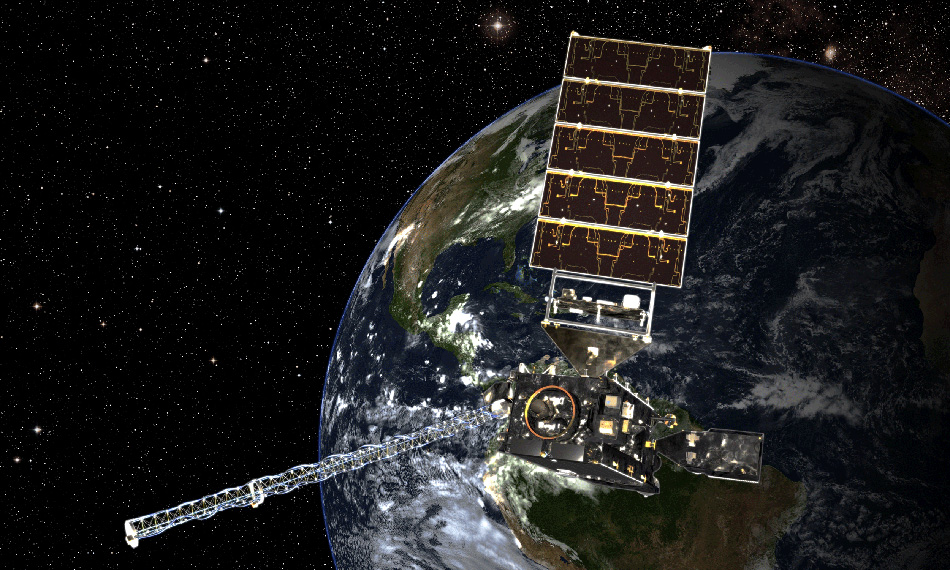
Improving Metal Polishing Technology
Coherent has been working to improve optical polishing technology for many years. We have developed our own, proprietary computer-controlled optical surfacing (CCOS) method and made major investments in advanced metrology equipment.
This enables us to polish metal mirrors with a shape accuracy that rivals that of glass optics. We reached this milestone some time ago, but our capabilities here still remain unique in the optics industry.
More recently, we’ve further refined our process to dramatically lower the surface roughness we get on metal mirrors. Now we can produce a surface smooth enough to be used as a mirror without any coating. But more commonly a thin layer (much less than one micron) of metal or dielectric material is applied to enhance the reflectivity. Gold is one commonly used coating material. Most importantly, these layers are thin enough to eliminate any issues related to a mismatch in CTE between the coating and mirror substrate.
The new generation of high-precision metal and composite mirrors possible with our technology makes these materials more viable and expands the telescope designer’s toolkit. Aluminum, in particular, becomes a more attractive option. It can reduce the cost and launch weight of optics without sacrificing image quality. And aluminum mirrors can usually be produced much faster than other materials which makes it easier to meet production schedules.
Learn more about Coherent custom telescope optics for space and terrestrial applications.