Quantum Dots and Photonics
The 2023 Nobel Prize in Chemistry spotlighted quantum dots, the innovative nanoscale photonic technology that’s already impacting diverse applications from oncology to next-generation displays.
December 19, 2023 by Coherent
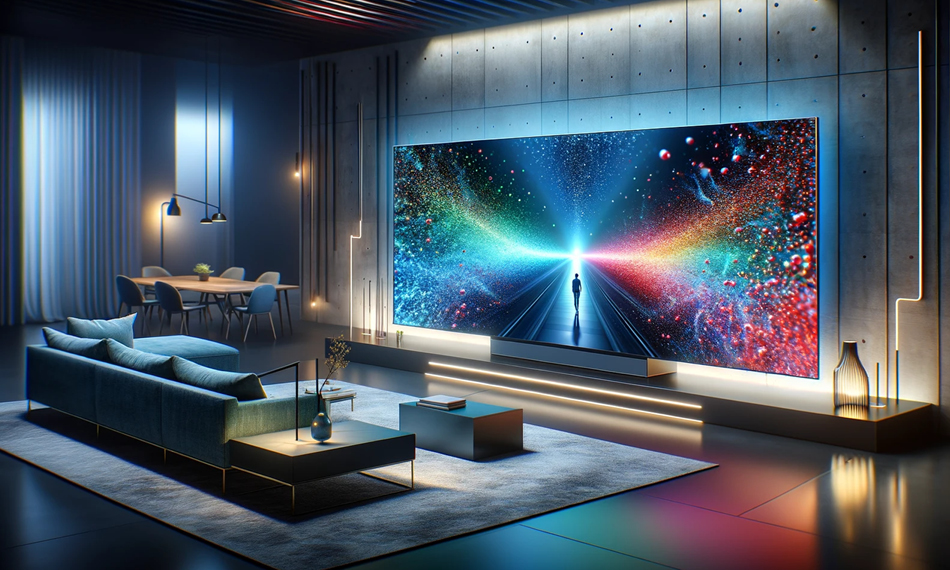
Today, we’re writing to celebrate the 2023 Nobel Prize in Chemistry, which went to Moungi Bawendi, Louis Brus, and Alexei Ekimov for their discovery and development of a unique new material: quantum dots.
Quantum dots are small particles of material with diameters of a few nanometers to a few tens of nanometers. They are called quantum dots because their small size means that the electrons in these tiny nanocrystals exhibit quantum behavior determined partly by the size of the particle, not just its chemical composition. Moreover, because they can be created in different sizes, this provides a method of producing materials with customizable electronic properties. And if the chosen material absorbs and/or emits light, this provides a way to make materials with customized photonic properties. Our lasers are often used to investigate and measure these photonic properties.
Because they provide customizable photonic behavior, quantum dots are already found in commercial products and used across many scientific disciplines, from physics and chemistry to medicine. Some of these products use lasers in their production and we will show an example that directly involves our lasers, but first let’s take a look at how quantum dots work.
How Quantum Dots Work - “Particle in a Box”
Quantum mechanics tells us that very small things like electrons behave not just as particles, but also as waves. And when waves are confined in some way – in a box if you like – the size of the box determines the size of the waves that are allowed. Larger boxes provide more space and support longer waves. Smaller boxes provide less space and only support shorter waves. In freshman college chemistry this simple theoretical model is called “particle in a box” even though it’s better described as “wave in a box.” Another simpler practical analogy is provided by sound waves created by a pipe organ. Longer pipes enable longer sound waves, which means a lower frequency that we then hear as a lower pitch. Shorter pipes can carry shorter sound waves, which means a higher frequency and higher pitch.
What does this mean for photonic properties of quantum dots? Well, if it’s a material that absorbs light such as cadmium sulfide, larger dots have similar absorption properties to the bulk material. But as the dots get smaller and smaller, the absorption profile shifts towards shorter wavelengths, i.e., towards the blue. Similarly, for materials that re-emit absorbed light as fluorescence, such as certain perovskite materials, large dots have similar emission properties to the bulk material. But as the dimensions of the dots get smaller, the emission is shifted to the blue.
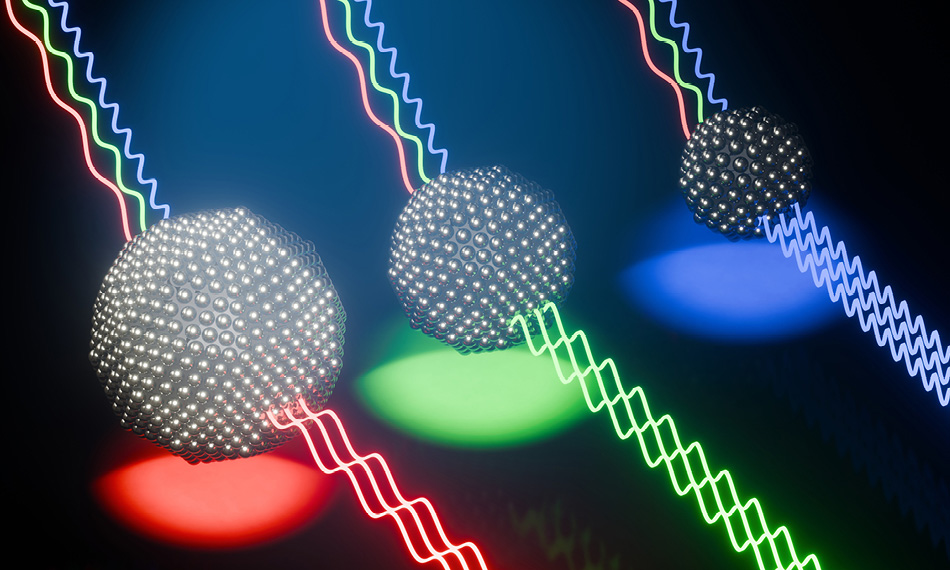
Quantum Dots and Display Technology
The ability to tune the light absorption and emission properties of materials by forming them as quantum dots of specific sizes means they can be used as color converters. These replace the role of old-fashioned phosphors in applications such as displays. We know a lot about display applications at Coherent, because our lasers are used in several important manufacturing processes, including for annealing the low-temperature polysilicon that often forms the backplane circuitry, for trimming the polarizer screens, and for mass transfer in the latest µLED displays.
Compared to traditional phosphors, quantum dots can deliver higher conversion efficiency and hence brighter displays. Plus their emission spectrum (color spread) can be quite narrow, enabling RGB displays with a larger color gamut.
Quantum dots have actually been used in television displays for nearly a decade in a format now commonly called QLED TVs. These are a type of LCD display where the dots are contained in a film, and the light that hits them is provided by an LED backlight. QLED TVs are currently some of the most popular TV types because they offer an attractive image quality for a lower cost than organic LED (OLED) displays, where each pixel is a light-emitting diode. There is also another TV variant called QD-OLED where quantum dots are again used as color converters/enhancers to enhance the picture color quality compared to OELDs alone.
An emerging display technology is called microLED. Here an inorganic LED is located in each pixel of the display, but it is much smaller than the pixel. This provides some important advantages. First, the microLED devices can be as small as a few microns so they can be produced densely packed on a wafer in enormous quantities and a very low unit price. And second, the large unused area of each pixel can be used for sensing or other purposes in future AR/VR applications. The tricky part of making these displays is to transfer and accurately place 100s of millions of the tiny LEDs in just minutes. Coherent provides a clever non-mechanical way of doing this that we call UVtransfer.
So where are quantum dots involved in microLED displays? It turns out there are already two types of microLED displays. In the original format, every pixel contains 3 LEDs – red, green, and blue. In another format, only blue LEDs are used with quantum dots acting as color converters for the red and green colors. This latter format addresses the limitations posed by the lower emission efficiency of red microLEDs.
Quantum Dots: Emerging Scientific Applications
Engineers and scientists in several other fields are using quantum dots for a variety of emerging applications, including some bioimaging methods for visualizing cancers. But quantum dots are still in their infancy and much basic research work remains to be done in terms of understanding how to optimize both the production and function of quantum dots in new material types. Our scientific and instrumentation laser products are used extensively in this work. Let’s look briefly at a couple of examples.
Pump-probe spectroscopy. Quantum dots emit light when electrons that have been excited to a higher energy – usually by absorbing light – release that energy by emitting fluorescence. But this process is never 100% efficient; some of the energy is lost in other processes. Scientists want to understand these processes to increase efficiency and other purposes. Ultrafast lasers are the best tools for these investigations because of the incredibly fast timescales involved. The lasers are often used in an approach called pump-probe spectroscopy where a femtosecond or picosecond laser pulse (called the pump pulse) excites the electrons and a second pulse (the probe pulse) then interrogates the sample in some way.
THz-Raman. All structures vibrate to some degree. The atoms in molecules vibrate at frequencies corresponding to infrared light, which is why you will find an infrared spectrometer in most chemistry labs. Nanoscale structures like quantum dots vibrate at terahertz (THz) frequencies. This is a tricky regime to investigate because THz radiation is hard to produce and hard to detect. Coherent has a simpler clever solution called THz-Raman that uses visible laser light to extract the THz information.
Some final thoughts
Nobel prizes in science are awarded to discoveries/inventions that reveal new science or that have major implications in terms of practical applications. In just a few years, quantum dots have proved to be excellent examples of both categories and represent an exciting area of photonics that is really only just getting started. As a company based in photonics, we are very happy to see that the developers have been honored by the Nobel Prize Committee with the 2023 Chemistry Prize. Truly well-deserved in our opinion!