GETTING THE BIG PICTURE IN AR/VR
Displays made with high refractive index waveguides provide a larger field-of-view, delivering a much more immersive user experience.
February 7, 2024 by Coherent
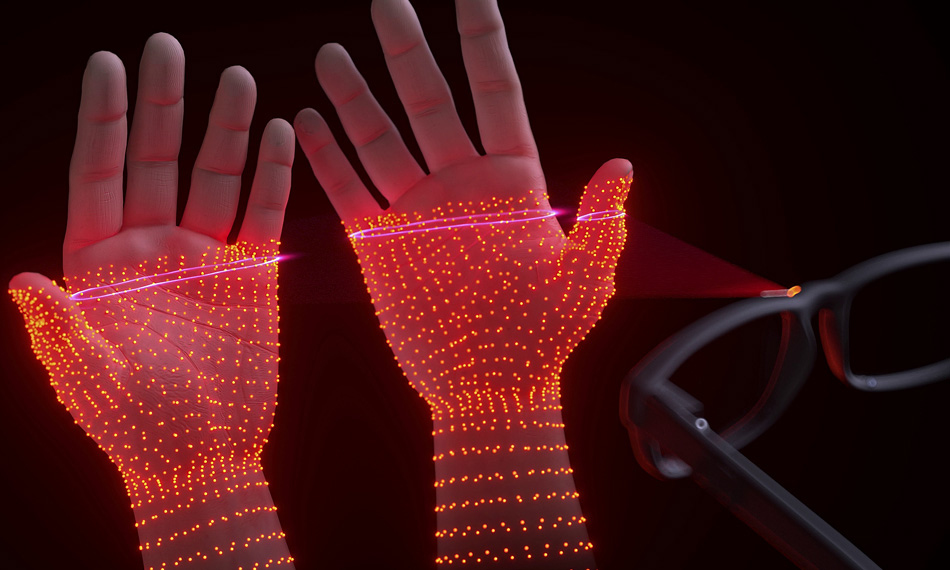
Remember the first cellphones that were the size of a brick, and about as easy to hold? Now, they’re sleek, powerful marvels that we all slip into a pocket or purse without a second thought and couldn’t live without.
Augmented reality (AR) goggles are poised to make a similar transformation, with the goal of becoming as comfortable and easy to wear as traditional eyeglasses. Advances in microprocessors, sensors, and connectivity are already combining to support this evolution.
But one of the most significant technological challenges still remaining for AR devices is the display itself. Specifically, the problem is creating displays that meet the exacting performance requirements of the human visual system, while still being small and lightweight. And, of course, which are economical to produce.
AR Headset Design Goals
Achieving all this requires AR display designers to meet several disparate goals at once. First the overall size, weight, and center-of-gravity of an AR goggle must make it comfortable enough to wear for extended periods of time.
Next, there are several important requirements for the display’s visual characteristics. We might just group some of these things together under the label of “crispness.” This includes properties like angular resolution and fill factor (the blank space between pixels). Color gamut and color accuracy are also considerations.
Plus, there’s the stereoscopic aspect of the display. Namely, it’s necessary that the apparent size, distance, and position of objects displayed by the headset match properly with the direct view of the real world. And the display needs to update fast enough as the wearer or external objects move.
The ease of fusing the stereoscopic image (which is created in the brain by the separate left and right eye views presented by the display) is also critical because problems with this almost immediately cause eyestrain and discomfort for most of the population. Just ask people what they think about 3D movies if you don’t believe this.
There are a few other key considerations around the concept of ‘immersiveness.’ Specifically, immersiveness increases as the display covers more of the wearer’s visual field. Technically, this is termed the display field-of-view (FOV). It’s also important to note that a consumer AR goggle needs to meet all these requirements for a population with a wide range of head sizes and eye separation distances (called interpupillary distance or IPD).
Waveguides Show Promise
As detailed in our previous post about AR technology, the particular challenge with AR headsets is that the display doesn’t sit directly in front of the viewer’s eyes. In contrast, in a VR headset, the viewer looks straight into the display, and optics are used to make it appear farther away and larger. But, optically speaking, this is a relatively simple task.
The AR headset optics must use a transparent component, called an “optical combiner,” which transmits light from the outside to allow the user a direct view of the real world. Plus, it must channel display engine output from the edge to the center of the combiner, and then redirect this towards the viewer’s eye. This is so that the computer-generated imagery appears overlaid on the real world view. This is a much more complicated task than performed by VR headset optics.
A wide variety of very clever optical systems have been developed to do this, and planar waveguides are one of the most promising technologies currently in use. A planar waveguide is like a tiny, transparent channel that guides light from a display engine to the viewer’s eyes. Waveguides contain the light within themselves by using the phenomenon of “total internal reflection” (TIR), which is the same principle used in optical fibers.
TIR occurs when light goes from a denser material (like glass) into a less dense medium (like air). When this happens, the light ray is refracted – it changes direction. Refraction is how lenses work.
But, if the light ray hits the boundary between the two materials at a large enough angle, it will be entirely reflected back; it won’t exit the material at all. The angle past which the light can’t exit the material is called the “critical angle.”
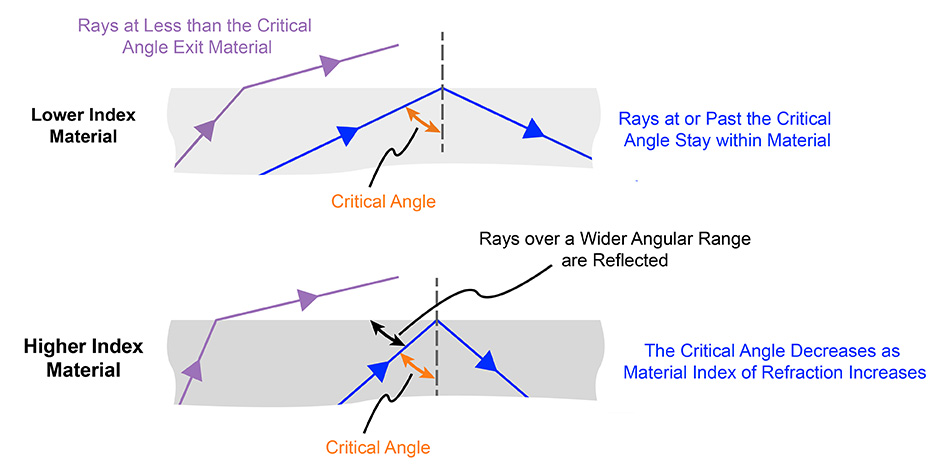
Light rays exiting a material into air are refracted (change direction). But, at larger incidense angles they are completely reflected back into the material and don’t escape at all. The higher the refractive index of the material, the smaller the angle at which this effect starts occurring.
To utilize this phenomenon in AR goggles, just imagine that an ‘in-coupler’ allows light from the display engine to be introduced into the waveguide at an angle greater than the critical angle. The light would then travel within this glass and be contained by TIR. At the center of the combiner, the light encounters an ‘out-coupler.’ This allows it to be extracted and directed towards the viewer’s eyes.
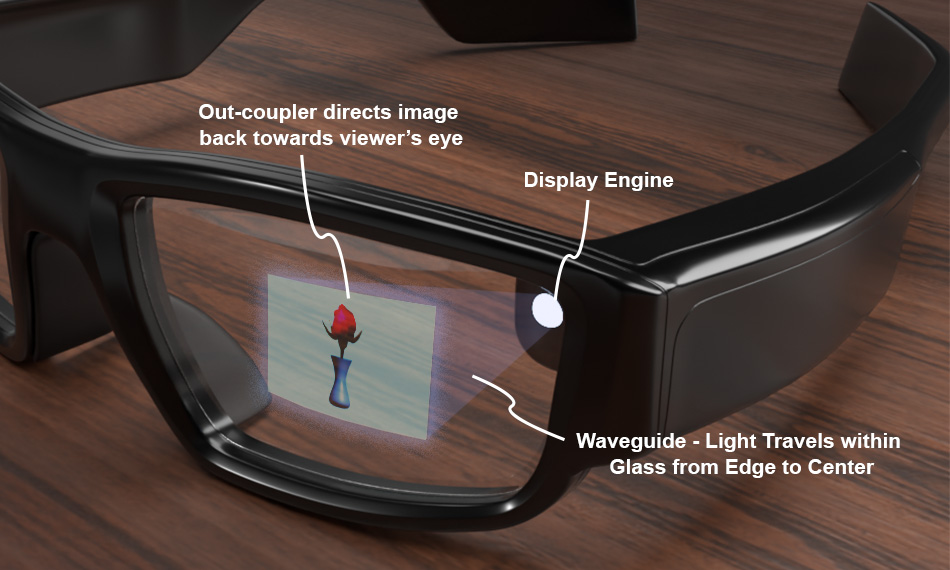
In a waveguide-based AR headset, light from the display is introduced into the waveguide near its edge using an in-coupler. It then travels through the waveguide using TIR and is coupled out when it reaches the point right in front of the viewer’s eye.
There’s a tremendous amount of technology and sophistication involved in actually making a waveguide like this work. But they do work and are already in use.
The benefit of waveguides is that they yield a headset that starts to look and feel very much like a regular pair of glasses. This brings us towards our goal of having a product sufficiently small, lightweight, and easy to use to gain widespread consumer acceptance.
Game-Changing Waveguide Materials
Waveguides work because of TIR, and there’s one important thing to know about that. Namely, as material refractive index increases, TIR occurs for light rays hitting the surface at smaller angles. This means they’re reflected over a wider angular range.
What this means is that using a higher refractive index material for the waveguide enables it to achieve a wider field-of-view. And FOV is key to producing the kind of immersive experience that AR system designers are striving to achieve.
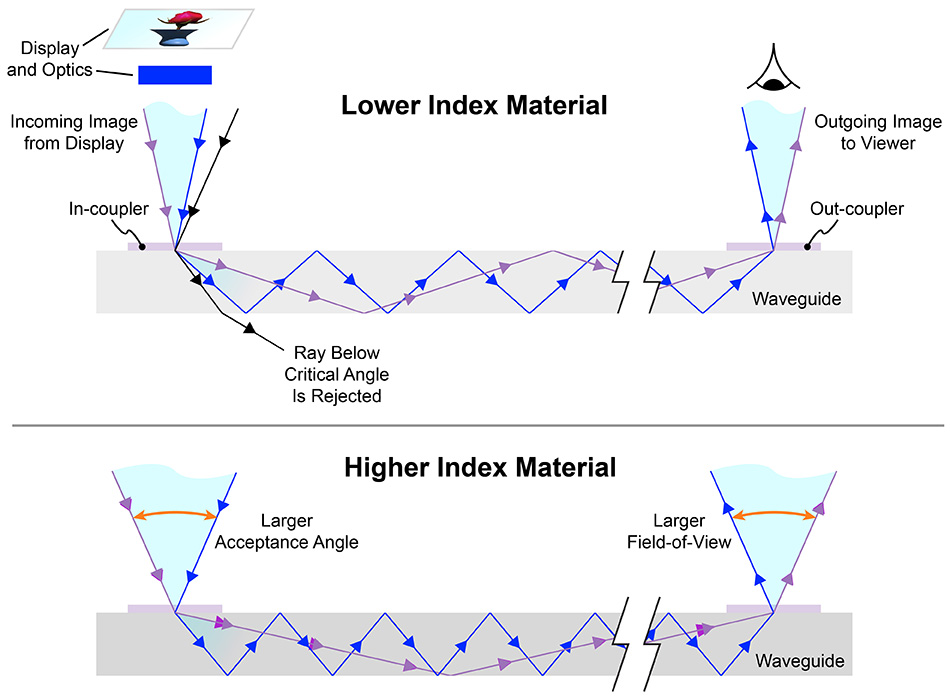
A waveguide made from a material with a higher index of refraction enables a larger field-of-view to be delivered to the viewer, which enhances immersiveness.
The problem is that refractive index of traditional optical glasses severely limits the FOV achievable with the kind of waveguide just described. Glass manufacturers have responded by developing higher index materials. And they’ve done an impressive job. But they can’t overcome the fundamental limitations of their materials. Right now, the highest refractive index achievable with glass is about 2.0.
But there are other materials besides glass that transmit visible light. And some of them have both higher refractive indices, as well as other desirable physical properties. Two of these are crystallline materials – lithium niobate (LiNbO₃), which has an index of 2.3 and silicon carbide (SiC), which has an index of 2.7.
The theoretical relationship between waveguide refractive index and display FOV is shown in the graph. SiC promises to essentially double the possible display FOV using even the highest index glass. This makes it a game-changer for the AR goggle designer.
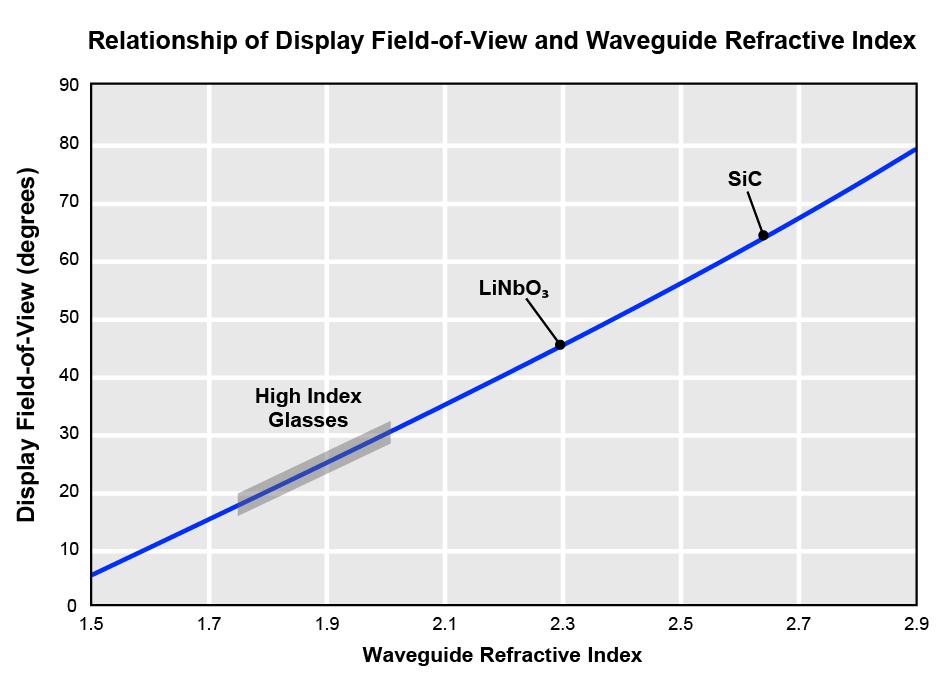
Theoretical relationship between waveguide material refractive index and maximum possible FOV of the AR display. Both LiNbO₃ and SiC offer huge gains over glass materials.
There’s another advantage of high index materials besides just larger FOV. Current waveguide designs often use either two or three separate glasses – one for each color (or one for two colors). The higher index of SiC, in particular, offers the possibility of combining all three color channels (red, green, and blue) into a single waveguide. This would provide a substantial improvement in headset size, weight, and cost. Plus, SiC is an exceptionally strong and lightweight material.
Both LiNbO₃ and SiC deliver practical and performance advantages over high-index glasses, but they are also more costly. On the other hand, their use can reduce overall system and manufacturing complexity which can lower production costs.
Coherent believes these materials can enable a new generation of AR devices with a compelling cost-benefit ratio for consumers. We’re already a vertically integrated manufacturer of both materials – from crystal growth through substrate fabrication. And, we can make other waveguide components, too, including diffractive couplers and optical coatings. Plus, all our manufacturing processes are scalable to large format and high volume. We’re ready to partner with AR system designers to develop waveguide displays based on these materials, and then reliably support them in volume production.