Buyer's Guide to Cutting Lasers
Many different types of lasers are used for diverse precision cutting tasks. Learn where each one of them excels.
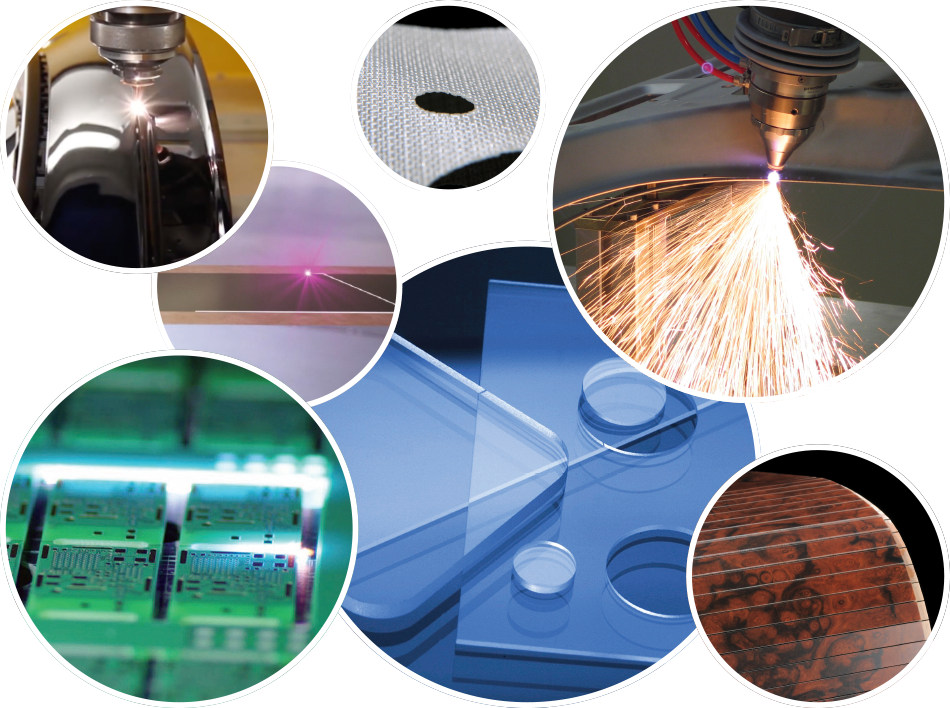
Buyer’s Guide to High-Precision Cutting Lasers
Learn to ask the right questions when choosing a cutting laser to get the capabilities, performance, reliability, cost, and support that will guarantee success in your application.
Table of Contents
- Why Use Lasers for Cutting?
- How to Use this Buyer's Guide
- Cutting Laser Overview Chart
- Laser Cutting Mechanisms
- Fusion Cutting
- Ablation Cutting
- Remote Cutting
- Cutting Laser Selection Checklist
- Process Factors
- Laser Factors
- Implementation Factors
- Selection Guide by Material
- Choosing a Laser Vendor
- Final Thoughts
- Ready to Get Started
Table of Contents
Why Use Lasers for Cutting?
Laser cutting offers numerous advantages over other methods of high precision cutting applications. These include:
- Non-contact processing which avoids damaging small or delicate parts.
- Zero tool wear, which lowers downtime and tool replacement costs.
- Highly consistent results.
- Unparalleled mechanical precision and the ability to produce fine details.
- Better edge quality and less debris than other methods which minimizes post-processing.
- Faster than other methods.
- Smaller kerf widths.
- Highly versatile, allowing easy reconfiguration to meet changing production requirements.
For all these reasons, lasers are used in an incredibly diverse range of precision cutting applications. They’re used for cutting thin glass and sapphire sheets for smartphones and tablets, composites, semiconductors, ceramics and more in advanced semiconductor chip packaging, nitinol, titanium, and steel in medical product manufacturing, and much more.
There are many types of lasers that service this broad spectrum of cutting applications. And sometimes laser manufacturers even create specific models that are optimized for a particular process. Choosing the right laser for your application from this tremendous selection of commercially available products can seem like an overwhelming task. Overwhelmed? Don’t worry. That’s exactly why we created this Buying Guide.
How to Use this Buyer’s Guide
The purpose of this Buyer’s Guide to Cutting Lasers is to help you select a cutting laser. We’re not going to tell you which laser to buy or which company to buy it from. However, we are going to provide you with a lot of context so you feel confident when you’re ready to make a purchase decision. More than anything else, our goal is to educate you on what questions to ask when you’re researching buying a cutting laser.
TIP: The Overview Chart provided lists the main types of precision cutting lasers currently available and is a great place to start your journey. Links embedded in the Overview Chart will take you to more information on the lasers themselves, or directly into the sections of this document that offer guidance on which of these technologies is usually most appropriate for a given application.
Getting this application specific information isn’t always straightforward because many vendors only offer a limited range of laser technologies. As a result, they promote what they have as being optimal for every use, whether it is or not.
Coherent is one of the world’s largest laser companies, and a global leader in materials and networking, as well. We service numerous cutting applications for medical devices, communications, microelectronics, instrumentation markets, and more. Most importantly, Coherent manufactures a comprehensive range of cutting lasers. This allows us to provide unbiased recommendations based solely on your unique needs and project requirements.
But what are your unique needs and project requirements? A critical first step in making an informed purchasing decision is properly identifying the considerations that are most significant in your own application. Some of the most common of these include:
- Technical factors, such as material compatibility, throughput speed, and cut quality
- Cost considerations , such as purchase price, maintenance costs, consumables, and operating costs
- Integrations factors, such as supported interfaces and communications protocols, and product size and weight
- Service, such as the geographic availability of spare parts and maintenance, and service response speed
- Applications support, such as vendor willingness to process samples, and aid in process development
A more detailed treatment of the typical considerations involved in cutting laser selection is provided in the Cutting Laser Selection Checklist .
Precision Cutting Laser Overview
This table lists the most commonly employed cutting lasers for high-precision applications, and provides a summary of their key characteristics and applications. Its purpose is to allow you to quickly identify the laser type(s) most likely to be useful for a specific application and therefore narrow your search.
Note: It shows the primary cutting mechanism for each laser type. Technical information on these processes is provided in the section on Laser Cutting Mechanisms.
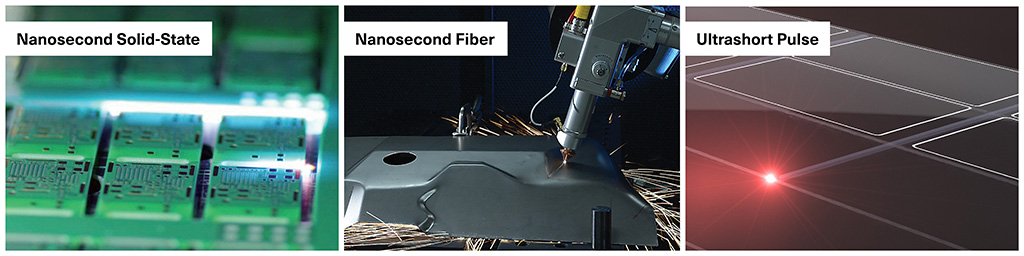
Cutting lasers comprise a wide range of wavelengths, pulse lengths, and output powers.
|
Nanosecond Solid-State Lasers |
Nanosecond Fiber Lasers |
Ultrashort Pulse (Picosecond/Femtosecond) Lasers |
|
Primary Cutting Mechanisms |
||||
Output Characteristics |
Average Power Range |
W – kW |
W – kW |
W – 100 W |
Wavelengths |
Infrared Green Ultraviolet |
Infrared Green Ultraviolet |
Infrared Green Ultraviolet |
|
Pulse Energy |
Higher, allowing deeper cuts per pulse, suitable for thick materials |
Lower, minimizing thermal damage, better for thin or heat-sensitive materials |
Typically lower pulse energy – but extremely high peak powers – enable cold ablation with minimal thermal damage |
|
Pulse Duration |
Longer pulse width, more heat diffusion into material |
Shorter pulse width, less heat diffusion into material |
Extremely short pulse durations virtually eliminate heat diffusion, resulting in "cold" material removal |
|
Peak Power |
Lower peak power, more material melting than vaporization |
Higher peak power, better for ablation and precision |
Extremely high peak power (gigawatts), enabling rapid vaporization without significant melting, ideal for ultra-precise ablation |
|
Pulse Repetition Rate |
Higher, leading to faster material removal but potential heat buildup |
Lower, reducing cumulative thermal effects, better for delicate materials |
Moderate to high repetition rates, depending on the system, allowing for fast and precise processing without significant heat buildup |
|
Beam Quality |
High beam quality with very tight focus, ideal for micromachining and ultra-precise cuts |
High beam quality with very tight focus, ideal for micromachining and ultra-precise cuts |
High beam quality with very tight focus, ideal for micromachining and ultra-precise cuts |
|
Practical Considerations |
Cutting Speed |
Faster due to high repetition rate and energy per pulse
|
Slower but more controlled, focused on precision
|
Fast but dependent on the material and pulse repetition rate, often slower for ultrahigh-precision tasks
|
Thermal Impact |
More thermal buildup, potential for larger heat-affected zone (HAZ) |
Lower thermal impact, smaller HAZ, cleaner cuts |
Minimal thermal impact; negligible heat-affected zone into material |
|
Maintenance |
Requires regular maintenance |
Minimal, robust, high uptime |
Generally low maintenance, but systems can be complex and costly to maintain |
|
Materials Compatibility |
Metals |
Metals |
Metals |
|
Key Takeaways |
Best for thicker metals, ceramics, and harder materials, and fast cutting (e.g., orthopedic implants, thicker semiconductors) |
Best for thin metals, polymers, semiconductors, and heat-sensitive materials, and high-precision cutting (e.g., stents, wafer dicing) |
Best for ultra-high precision tasks like cutting semiconductors, thin films, glass, polymers, or advanced medical devices where heat damage must be avoided and when no post-processing desired |
Laser Cutting Mechanisms
All solid substances are held together by bonds or attractive forces between the atoms, ions, or molecules that compose the material. At the most basic level, cutting any solid substance requires breaking those bonds.
In traditional mechanical cutting, such as with a saw or knife, the cutting tool applies force to the material over an area concentrated around the tool edge. This creates a shear which stretches the bonds between those particles being subjected to the force and neighboring ones which are not. If the force is sufficiently strong, the bonds will break. This is the fundamental physical process that occurs whether it’s cutting paper with scissors, sawing lumber, or carving a roast.
Lasers are non-contact tools. They do not impart physical force to objects they illuminate. Instead, they cut using entirely different mechanisms. However, they must still accomplish the same end result, namely, breaking atomic or molecular bonds over a contiguous region to produce a cut.
Laser cutting can be split into the four main categories:
Fusion laser cutting
Ablation laser cutting
Remote laser cutting
Reactive or flame laser cutting
Reactive or flame laser cutting isn’t generally used for high-precision applications. The other three methods are detailed here.
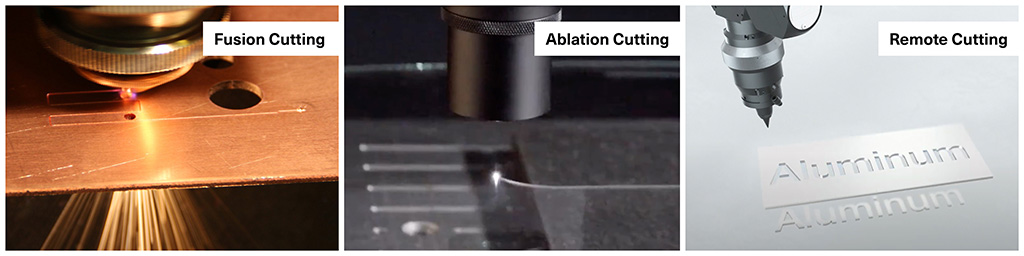
Basic mechanisms of laser cutting.
Fusion Cutting
In fusion cutting, the laser heats the target material to the point of melting, creating a molten pool. Simultaneously, a high-pressure assist gas (typically nitrogen or, in some cases, argon) is directed coaxially with the laser beam to expel the molten material from the cut region. The assist gas also helps cool the cut zone, preventing oxidation and ensuring a cleaner edge. This method offers remarkable precision and is ideal for creating intricate, burr-free cuts in metals, ceramics, and certain polymers.
One of the key advantages of fusion cutting is its ability to maintain fine tolerances and achieve smooth, high-quality edges, which is critical for components like stents, surgical tools, or microelectronics. These applications demand not only dimensional accuracy but also minimal thermal damage to the surrounding material, as any excess heat can degrade performance or require costly post-processing.
Fusion cutting is particularly well-suited for materials like stainless steel, titanium, and silicon wafers, where the use of a non-reactive assist gas like nitrogen ensures a clean cut without compromising the integrity of the material. With gas pressures ranging from 75 to 250 psi, fusion cutting can handle thin or thick materials, depending on the specific requirements of the application. Its precision, combined with the ability to cut complex geometries, makes fusion cutting a cornerstone for industries where precision, cleanliness, and efficiency are paramount.
Ablation Cutting
Ultrashort pulse (picosecond and femtosecond) lasers offer a unique cutting method due to their extremely short pulse duration and very high (gigawatt level) peak powers. As the pulse duration is shorter than the “conduction time” of materials, the high peak power causes extremely rapid, localized heating that transforms the solid material to vapor instantaneously. The vapor is blown through the cut with a coaxial assist gas, typically argon. This ablation cutting method creates the highest possible quality cut edges, with high dimensional accuracy and no burrs. The absence of burrs is highly significant for applications such cutting nitinol tubes for the medical device industry, and stainless steel or titanium for the luxury watch industry, as the cuts require little to no post processing.
Remote Cutting
The key difference between remote cutting and the other methods is that the laser head is located far from the work surface. This allows the focused laser beam to be moved at high speed (>33"/s, 1 m/s) across the surface by a scan head. No coaxial assist gas is used. Depending on the material thickness, the scan head may trace the same exact cut path multiple times to create a through cut.
The cutting mechanism itself occurs through melt ejection; the laser melts, but also vaporizes, a fraction of the metal, and the subsequent vapor expansion pressure forces the melt out of the cut. Remote cutting paths are usually simple – such as circles or squares – to facilitate high cutting speeds.
Remote cutting is used for mostly thin materials, such as battery foil cutting or thin plastic films of less than 0.01" (0.25 mm) thickness. Laser sources must have sufficient intensity to drive the vaporization cutting mechanism.
Cutting Laser Selection Checklist
These tables are your roadmap to selecting the right cutting laser. They list some of the key factors that can impact your choice and will help prompt you to identify what truly matters for your specific application. Use them to formulate the questions to ask when qualifying a particular laser type and specific vendor.
Process Factors
Laser selection almost always begins by first identifying and defining the process requirements and the desired outcome.
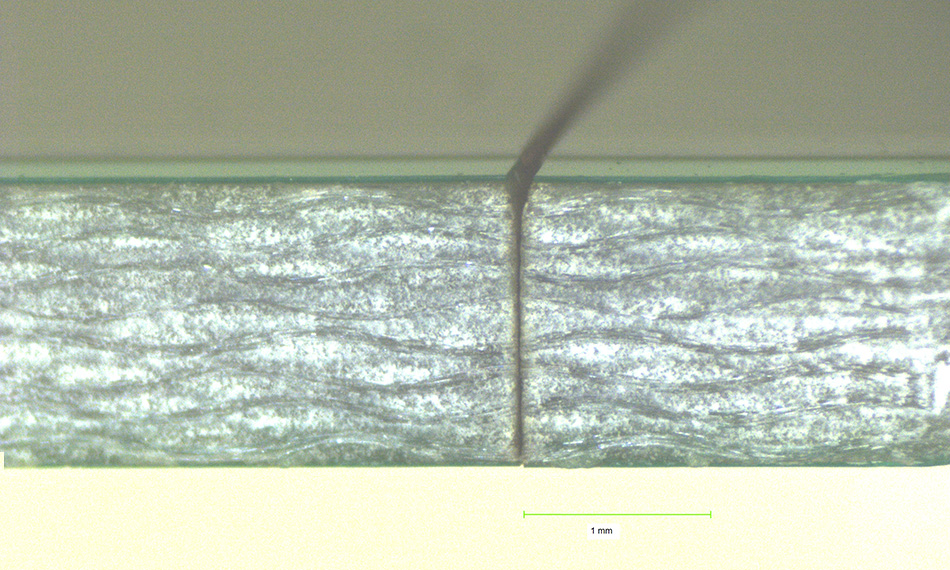
Kerf width is often an important consideration in laser cutting.
Factor | Notes |
Material Type |
Material characteristics usually play the largest role in determining the appropriate cutting laser. The linear absorption properties of the material (what wavelengths it absorbs), reflectivity, thermal conductivity, and melting point are generally the properties of greatest importance, particularly when using nanosecond solid-state and nanosecond fiber lasers. The Overview Chart offers a good starting point for matching laser to material. |
Material Thickness |
Material thickness and the desired cutting speed usually dictate the necessary laser power. Thicker materials generally require higher power and slower cutting speeds to ensure clean cuts. |
Required Speed |
There is often a trade-off between cutting speed and cut quality. Higher speeds may decrease precision, so balance speed with the desired cut quality. |
Heat-Affected Zone (HAZ) |
Minimizing the HAZ is critical for heat sensitive materials and small parts. USP lasers provide the minimum HAZ achievable. |
Kerf/Edge Quality |
Certain laser/material combinations tend to deliver smoother edged cuts and less microcracking. These can both affect the subsequent mechanical strength of the cut part. Smoother edges can also reduce the need for post-processing. Keep in mind that the polarization of the beam relative to the cutting direction can have a big effect on kerf quality. |
Debris Production |
High amounts of debris can increase cleanup time and necessitate more post-processing steps. |
Post-Processing |
The need (or lack thereof) of post-processing can dramatically impact overall process cost and throughput. Certain types of post-processing may also have a significant environmental impact. |
Laser Factors
With the process requirements defined, the next step is typically selecting the right laser technology. In many cases, the technical requirements of the process – the material, its thickness, the desired throughput, and so on – narrow the search to a single laser type.
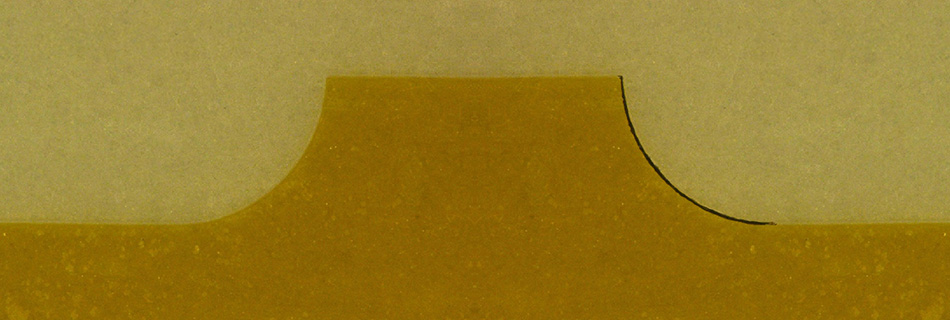
Heat-affected zone (HAZ) is another concern in many cutting applications.
Factor | Notes |
Output Power |
A certain minimum power is often required to cut a given material and thickness. Increasing the power above this threshold level raises throughput. It may also increase cost, since higher laser power generally costs more money. In some situations, there may be an optimum power, and exceeding this level might have negative consequences, such as a larger HAZ, reduced cut quality, or increased debris production. These are some of the reasons why, for both nanosecond solid-state and USP lasers, it’s common to use “multi-pass” cutting. That is, the laser scribes over the same area repeatedly to produce a through cut. |
Wavelength |
Laser wavelength is a primary factor for two reasons. First, matching laser wavelength to material absorption optimizes cutting efficiency and speed, and often cut quality. The Overview Chart summarizes laser compatibility with common materials. Second, shorter wavelengths typically enable greater cut precision, since they can be focused to smaller spot sizes. Depending upon the beam delivery optics, shorter wavelength may also allow greater depth of focus, which enhances process stability. |
Beam Quality (M² or BPP) |
Beam quality determines how well the laser can be focused. This has two significant consequences. First, a smaller focused spot (lower M² or BPP) enables smaller kerf width cuts and the production of finer, more detailed features. Second, for a given laser power, a higher power density can be achieved in the focused spot as beam quality improves. Higher power density means more energy is delivered within a specific area, which enhances the ability to melt or vaporize the material efficiently. Better beam quality typically makes the overall power of the laser more useable. |
Output Mode (Pulsed or CW) |
The choice between a pulsed or continuous wave (CW) laser is highly dependent on material and desired results. Furthermore, some laser types will only operate in one of those modes. This makes it difficult to generalize. But, overall, CW lasers are usually preferred for high-speed, high-throughput applications involving thick or dense materials. Typical applications are cutting thick metal in automotive and energy applications. Pulsed lasers generally excel in providing higher precision cutting, minimal thermal impact, and processing of specialized materials. They’re often used for cutting delicate or heat-sensitive polymers, ceramics, and thin metals in emobility, semiconductor, and medical device manufacturing. |
Beam Delivery |
Beam delivery options, such as fiber or free-space optics, significantly impact the practical aspects of configuring a laser cutting system, as well as its associated costs. Fiber delivery offers tremendous convenience and flexibility, making it easy to integrate a cutting laser into a space-constrained production environment alongside other equipment. It also simplifies maintenance by making the laser more accessible. CO₂ lasers cannot utilize fiber delivery, resulting in more complex beam delivery systems that can be subject to misalignment over time. |
Operational Consistency |
Laser output variations can lead to inconsistent cuts, affecting product quality and increasing scrap rates or the need for rework. Lasers which operate with consistent output characteristics reduce maintenance downtime, ensuring continuous operation and productivity. Especially as production volumes increase, it’s critical to know how the laser manufacturer has built in and ensures operational consistency. |
Cooling Requirements |
Lasers often require cooling systems which utilize air or water. Water cooling may need a specific infrastructure within your facility to support it. This can have a cost associated with it. |
Operating Cost |
Many cutting lasers use consumables such as gases (especially assist gasses), optics, and protective windows. Different laser types also have their own maintenance requirements and reliability characteristics. And, of course, these can vary by manufacturer. Maintenance downtime in a high-volume production environment – both scheduled and unplanned – often represents a larger cost than the original purchase price of the laser itself. This makes it very important to establish realistic expectations about laser uptime and reliability and understand manufacturers’ warranties and lifetime specifications. |
Implementation Factors
Many laser manufacturers offer similar products – or at least products that appear to be similar. To choose one, practical considerations related to cost, integration factors, applications development, and ongoing support must be considered. These factors are frequently the determining factor in selecting a specific vendor.
Factor | Notes |
Applications Development |
Vendor support in developing and optimizing your cutting process can be invaluable in many cases. If so, look for laser vendors that offer sample processing and process development assistance. |
Purchase Cost |
Consider the capital cost of the laser, plus installation and setup costs. Staff training may also be a cost factor. |
Operating Costs |
Evaluate ongoing expenses such as maintenance, consumables, and energy usage. Some lasers have inherently lower running costs which can save money over time. Downtime is important to consider, since it may represent a larger risk factor and cost than the capital cost of the laser. |
Service & Support |
Evaluate the geographical availability and typical response time for service and support for potential vendors. Consider the geographical availability of spare parts to ensure minimal downtime in case of repairs or maintenance. |
Software |
User-friendly and versatile control software can significantly enhance productivity and ease-of-use. Ensure the software is compatible with your existing systems. |
Integration |
The ease of integrating the laser with your existing production line or machinery is crucial. Look for lasers with flexible interfacing options and comprehensive integration support, and make sure your specific integration and communications protocols are supported. |
Ease-of-Use |
A user-friendly human-machine interface (HMI) reduces training time and operational errors. Prioritize systems that offer intuitive controls and clear operational guidance. Determine if the laser vendor offers training. |
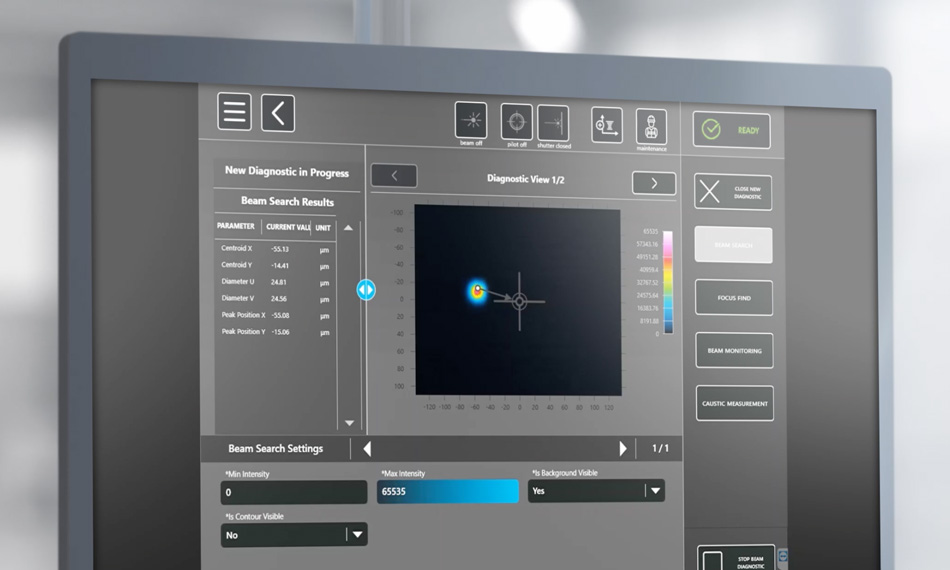
The human-machine interface (HMI) often determines the real world productivity of a laser cutting system.
Selection Guide by Material
The selection of a specific cutting laser technology is almost always driven by the material – its absorption characteristics and thickness, together with factors related to cutting speed and kerf quality. This table lists some common materials and the lasers typically used to cut them.
Material Class | Example Lasers | Main Characteristics | Comments |
Metals |
Nanosecond Fiber |
High power density enables rapid cutting and the ability to process thicker materials. |
Particularly useful for cutting thin to medium thickness metals, offering a good balance of speed and precision. The availability of green and UV outputs further enhances their ability to handle reflective metals like copper and aluminum, minimizing heat-affected zones and ensuring clean cuts. |
Provides precision for intricate cuts on thinner substrates. |
Ideal for precision cutting of thin metal substrates where high accuracy is required. With green (532 nm) and UV (355 nm) wavelengths available, they offer enhanced absorption for reflective metals, reducing thermal distortion and improving edge quality. However, they are generally less efficient than fiber lasers for thicker metals, where fiber lasers perform better. |
||
When the highest precision is required, or for very delicate parts. |
Lower throughput rates usually limit these lasers to the most demanding, precise, and heat-sensitive applications. |
||
Polymers |
Nanosecond Fiber |
Effective for cutting polymers with high absorption at the fiber laser wavelength (typically 1064 nm), but thermal effects such as melting and discoloration are more pronounced compared to ultrashort pulse lasers. Fiber lasers are best used for thicker or less heat-sensitive polymers. |
Potential for melting and burning – varies by material. |
Precise, clean cuts with minimal thermal distortion and HAZ. |
The combination of edge quality and minimal HAZ makes them particularly useful in microelectronics and medical device manufacturing. |
||
Glass |
Nanosecond Fiber |
Efficient cutting with moderate heat-affected zones, but generally requires careful handling to avoid chipping. |
Commonly used for industrial applications where speed is important but precision cutting of thin glass is also needed. |
Produces chips in the single digit micron size range and can cut any shape. |
Usually performed with green (532 nm) or ultraviolet (355 nm) lasers in “bottom-up” cutting – here the laser enters through the top of the transparent substrate and is initially focused on the bottom surface. |
||
Incredibly high precision possible, as well as the ability to cut any shape, including cut-outs. Chips are in hundreds of nanometers in size range, with often eliminates post-processing. |
Usually uses infrared (1064 nm) and some form of “filamentation” cutting like Coherent SmartCleave. |
||
Ceramics |
Nanosecond Fiber |
Can be used for cutting ceramic materials with good speed and precision, though thermal effects can be more pronounced than ultrashort pulse lasers. |
Used when a balance of speed and precision is needed in ceramics cutting, such as for tiles or advanced ceramics. |
Uses near-infrared, green, or ultraviolet lasers depending upon the material, required precision, and desired HAZ. |
Provides a good balance between cutting speed and precision. |
||
Highest precision and smallest HAZ but slowest cutting speed. Can use near-infrared, green, or ultraviolet lasers. |
Useful where minimal thermal impact is crucial, such as in medical devices, microelectronics, and advanced manufacturing. |
||
Semiconductors |
Nanosecond Fiber | Used for moderate precision tasks where speed is a priority and the material thickness allows some thermal effects. | Suitable for dicing thicker semiconductor wafers, where speed is more important than minimizing heat effects. |
Uses near-infrared, green, or ultraviolet lasers depending upon the material, required precision, and desired HAZ. |
Provides a good balance between cutting speed and precision. |
||
Highest precision and smallest HAZ but slowest cutting speed. Can use near-infrared, green, or ultraviolet lasers. |
Ideal for cutting thin substrates or creating intricate patterns without causing thermal damage. |
||
Composites | Uses near-infrared, green, or ultraviolet lasers depending upon the material, required precision, and desired HAZ. |
Effective for detailed and intricate cutting of composite materials used in automotive, aerospace, and industrial applications. |
|
Highest precision and smallest HAZ but slowest cutting speed. Can use near-infrared, green, or ultraviolet lasers. |
High-precision cutting of composites where minimal thermal impact is crucial, such as in aerospace and medical applications. |
Choosing a Laser Vendor
Several factors beyond just the laser/material interaction play a vital role in the overall success and efficiency of a laser cutting system in an actual production environment. These include integration capabilities, service and support, application assistance, and more. These elements can impact the laser system’s capital cost, operating expenses, downtime, required training for operating and maintenance personnel, and much more.
Here are some key factors to consider when evaluating potential laser vendors:
Integration Capabilities
1. Supported Interfaces and Communication Protocols
- Importance: Ensuring that the laser system can seamlessly integrate with your existing production line and equipment is crucial. Compatibility with your control systems and communication protocols can significantly affect the ease of integration and operation.
Considerations: Check if the vendor supports common industrial communication protocols such as Ethernet, Modbus, PROFINET, and others.
2. Product Size and Weight
Importance: The physical size and weight of the laser system can impact how it fits into your existing production environment or how easily it can be integrated into a tool, such as on a robot arm.
Considerations: Evaluate the footprint of the laser system and ensure it fits within your workspace. Consider the weight if the system needs to be mounted or installed in a specific location. Compact and lightweight systems can be advantageous in space-constrained environments.
3. Ease-of-Use
- Importance: A user-friendly HMI and overall ease-of-use are critical for efficient operation to reduce training time, and to minimize operator errors. An intuitive interface can help operators quickly learn and effectively manage the laser system and implement changeovers in production with minimal downtime.
Considerations: Assess the HMI for ease of navigation, clarity of information, and accessibility of controls. Look for systems that offer customizable interfaces and straightforward operational procedures. The availability of training and support from the vendor can also enhance ease-of-use.
Service and Support
1. Geographic Availability of Spare Parts and Maintenance
Importance: Having readily available spare parts and maintenance services can minimize downtime and ensure continuous operation. The location of the vendor’s service centers and parts depots can significantly affect response times.
Considerations: Check if the vendor has a robust supply chain and service network in your region. The proximity of service centers and availability of spare parts can reduce lead times for repairs and maintenance.
2. Service Response Speed
- Importance: Quick response times for service and maintenance issues are critical to maintaining high production uptime. Delays in service can lead to significant production losses and increased operational costs.
Considerations: Evaluate the vendor’s average response times and their ability to provide on-site support. Consider service agreements that guarantee response times and prioritize vendors with a proven track record of prompt service.
3. Reliability and Warranty
- Importance: The reliability of the laser system and the warranty offered by the vendor can impact long-term operational costs and confidence in the system.
Considerations: Investigate the reliability ratings of the vendor’s laser systems and the terms of the warranty. A comprehensive warranty can provide peace of mind and protect against unforeseen expenses.
Applications Support
1. Vendor Willingness to Process Samples
- Importance: The ability of a vendor to process samples of your material can provide valuable insights into the laser’s performance and suitability for your specific application. This also demonstrates the vendor’s commitment to supporting your needs.
Considerations: Look for vendors who offer sample processing as part of their pre-sales support. This allows you to evaluate cut quality, speed, and other critical parameters before making a purchase decision.
2. Process Development Support
- Importance: Vendors that assist in developing and optimizing your cutting processes can significantly enhance your production efficiency and quality. This support can be invaluable, especially for complex or new applications.
- Considerations: Choose vendors that offer process development support, including the optimization of cutting parameters and integration of the laser system into your production line. Access to expert advice, experience, and technical support can help you achieve the best results.
3. Training and Documentation
- Importance: Proper training and comprehensive documentation are essential for the smooth operation and maintenance of the laser system. Skilled operators can maximize the system’s capabilities and minimize downtime.
Considerations: Ensure the vendor provides thorough training programs for your staff, including initial training and ongoing education. Comprehensive documentation, including user manuals, maintenance guides, and troubleshooting tips, is also crucial.
Overall Vendor Reputation
1. Industry Experience and Expertise
Importance: A vendor’s experience and expertise in the laser cutting industry can provide confidence in their ability to meet your specific needs and challenges.
Considerations: Research the vendor’s history, customer base, and the range of applications they have supported. Vendors with a broad portfolio and a strong reputation are often better equipped to handle diverse and complex requirements.
2. Customer Reviews and Testimonials
- Importance: Feedback from other customers can provide valuable insights into the vendor’s performance, reliability, and support quality.
Considerations: Look for customer reviews, case studies, and testimonials that highlight the vendor’s strengths and areas for improvement. Positive feedback from similar industries or applications can be particularly reassuring.
Choosing the right laser vendor involves evaluating various factors beyond just the technical specifications of the laser system. By considering integration capabilities, service and support, application assistance, and the vendor’s overall reputation, you can make a more informed decision that ensures long-term success and efficiency in your laser cutting operations. Make sure to conduct thorough research and engage with potential vendors to address all your concerns and requirements.
Final Thoughts
Laser cutting technology offers unparalleled precision, versatility, and efficiency, making it a valuable tool in supporting some of the most important trends common across many high technology industries. These include greater miniaturization, increased functionality, improved reliability, and lower cost.
This Buyer’s Guide to Cutting Lasers provides an overview to help you navigate the complexities of selecting the right laser for your specific application. By understanding the different types of lasers, their cutting mechanisms, and the trade-offs involved, you can make informed decisions that optimize your production processes.
But in addition to picking the best type of laser, it’s also important to choose the right laser vendor. Especially in volume production settings or demanding applications, this choice can have a substantial cost impact.
So, look for a vendor who offers comprehensive support. This starts with expertise in applications development – this can make the difference between a process that consistently delivers high-quality parts and one that has you battling scrap rates and rework. And it extends to responsive service to get you running again when problems do occur.
This guide aims to empower you with the knowledge to ask the right questions and identify the most significant factors for your needs. By leveraging this information, you can ensure that your laser cutting operations are efficient, reliable, and cost-effective, driving success in your applications and contributing to the overall growth and innovation of your business.
Ready to take the next step? Talk to a Coherent Laser specialist.
Contact Sales
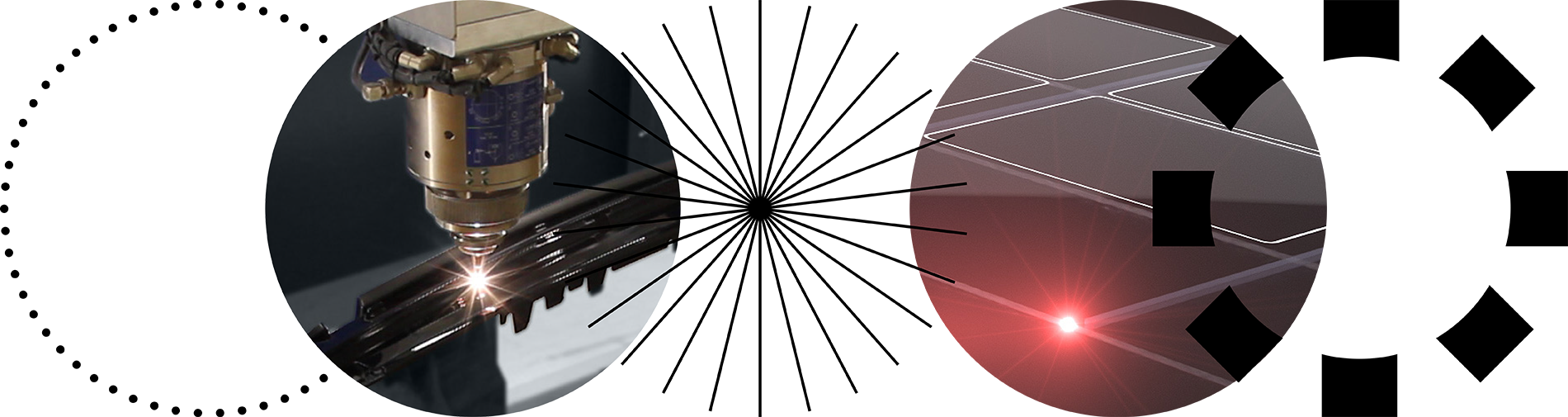