WHITE PAPER
OPSL Advantages Whitepaper Series #2:
Invariant Beam Properties
Overview
The optically pumped semiconductor laser (OPSL) is a unique patented technology that combines the most desirable attributes of laser diodes, DPSS (Diode Pumped Solid State) and Ion lasers, while eliminating a number of their compromising limitations. One of their key advantages is the freedom to adjust the output power over a large range (10-100%) with no effect on important output beam parameters, including beam divergence, beam shape and beam pointing.
OPSL Advantages White Papers in this series:
#1. Wavelength flexibility
#2. Invariant beam properties
#3. No mode noise ("green noise")
#4. Superior reliability - huge installed base
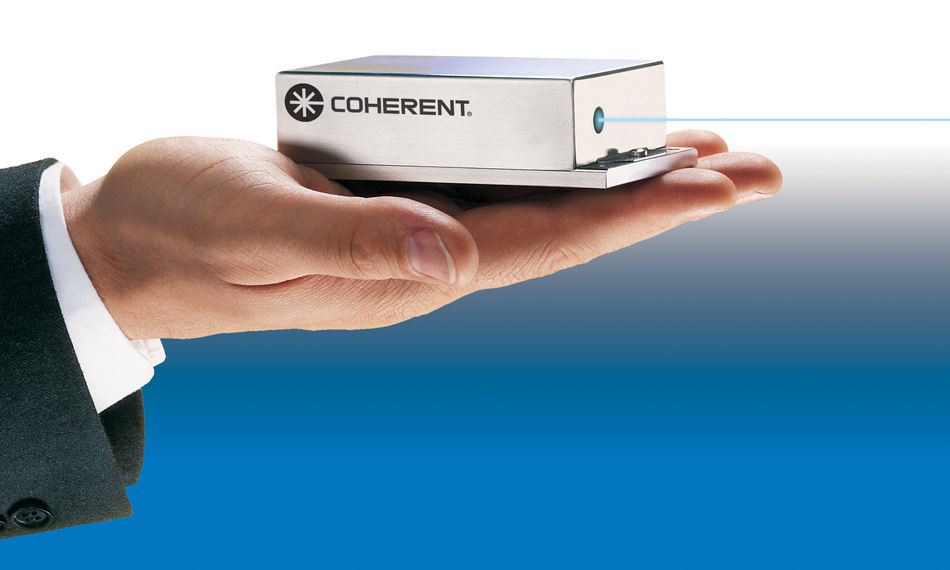
The Advantages of Independent Power Adjustment
The ability to vary laser output power or to operate a laser at less than its maximum power is often an important capability. In many instances, using the “power knob” is essential to optimize processes or experiments like preventing detector saturation or sample damage. It is also often preferable to perform system alignment and testing with reduced power – to minimize the risk of damage and to maximize eye safety. Also superresolution microscopy techniques like STED require fine power adjustment to optimize nanometer scale resolution. Thus, the option to smoothly reduce output power is more desirable than resorting to some type of attenuator, both for ease of use and set-up simplicity.
Unfortunately, in most other solid state lasers, reducing power from the manufacturer’s specified optimum value also compromises beam properties: in particular, beam divergence, and also beam diameter, mode quality and beam pointing. The reason is a phenomenon called thermal lensing that is common to solid state lasers based on bulk materials such as Nd:YVO4.
The Problem of Thermal Lensing
When a laser gain crystal or glass is optically pumped, some of the pump power is unavoidably converted to heat. In addition, self-reabsorption of the laser beam heats the active volume of the crystal. In order to stabilize performance and avoid damage, the gain crystal is cooled in some way. This can take the form of a passive heat sink, water cooling, thermoelectric (TE) cooling and even cryogenic cooling. Irrespective of the type of cooling, heat removal takes place through one or more of the crystal surfaces. Under steady state operation, this sets up a thermal gradient in the gain crystal.
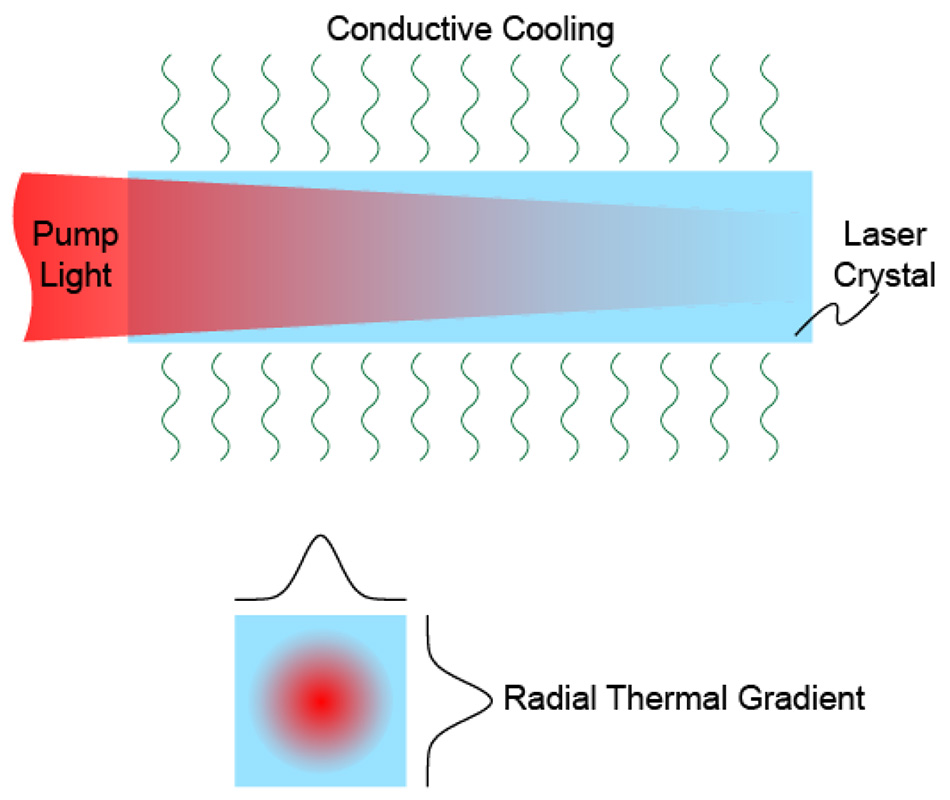
Figure 1: In a laser based on optical pumping of a bulk crystal, the pump light causes an unwanted radial thermal gradient and often a longitudinal gradient as well, leading to strong thermal lensing with a lens power that varies with changes in the pump power.
There are two consequences of this thermal gradient. Firstly, the refractive index changes according to the temperature distribution in the laser medium. In addition, the crystal expands as it heats up, producing a change in the curvature of its optical surfaces. In the simplest case of an end-pumped cylindrical laser rod, these effects create a spherical lens whose power is proportional to the length of the crystal and the pump power. Moreover, the lens power may also be affected by any longitudinal gradient, particularly if the gain crystal is only pumped from one end.
Optimizing the output power in a high quality Gaussian beam profile (TEM00) requires a careful design of the resonator, inclusive of the best possible spatial match of the laser mode with the pumped volume. Any change of the curvature of optical surfaces or – equivalently – spatial gradients of the refractive indices during operations will result in suboptimal mode quality or efficiency because of these “thermal lensing” effects. Of course the degree of this thermal lens depends on the pump power applied to the laser medium.
In solid state lasers, thermal lensing will change the output beam divergence and diameter. In high-performance lasers like Coherent’s AVIA™ family of industrial DPSS lasers for example, a feedback feature called ThermaTrak™ addresses this problem by moving a motorized intracavity lens as the power is adjusted. Conversely, in lower-performance DPSS lasers, the thermal lens is not controlled and its variations as the pump power is changed will result in beam parameter changes, reduction in efficiency and limited usable power range. Since most commercial DPSS lasers do not include variable compensation, their output beam parameters are only guaranteed at the specified output power.
OPSL – Thin Gain Chip – No Thermal Lens
In an OPSL, the gain medium is a very thin (< 10 μm) disk of semiconductor quantum wells overlaying dielectric layers that act as a rear surface total reflector. The rear surface in turn is bonded to an actively cooled heat sink, efficiently cooling the semiconductor structure. Although a radial thermal gradient still results from laser operation, the entire structure is so thin that thermal lensing is negligible; in fact the path length in the gain material is approximately 1000 times less than in a typical DPSS.
To confirm the assumption of negligible thermal lensing, Coherent engineers performed a series of tests to monitor the optical properties of an OPSL gain chip in which a thermal gradient was deliberately created and interferometrically measured. Moreover the test gradient was designed to be significantly larger than any that could be developed under normal laser operation, even at full OPSL output power.
Figure 2 shows the setup used in these tests. Here an OPSL laser cavity was modified with wedged beamsplitters enabling a test beam to probe the OPSL chip as it is simultaneously irradiated by varying amounts of pump power. Specifically, a coherent single-mode laser beam at 980 nm is split by the first beamsplitter so that part of its intensity is reflected off the OPSL chip and part is reflected from a super-flat reference mirror. A second beamsplitter recombines these reflected beams in a configuration called a Mach-Zehnder interferometer. The recombined beam is then expanded and viewed using a CCD camera.
If the OPSL chip remains flat with no thermal lensing, then the image at the camera would be uniform across its profile. Conversely, any thermal lensing will show up as dark and light interference fringes whose spacing quantitatively measures the extent of any lensing or other beam distortion. Careful evaluation of this test rig with a heated mirror in place of the OPSL gain chip showed it could discern wavelength distortions as small as λ/50 at the 980 nm test wavelength.
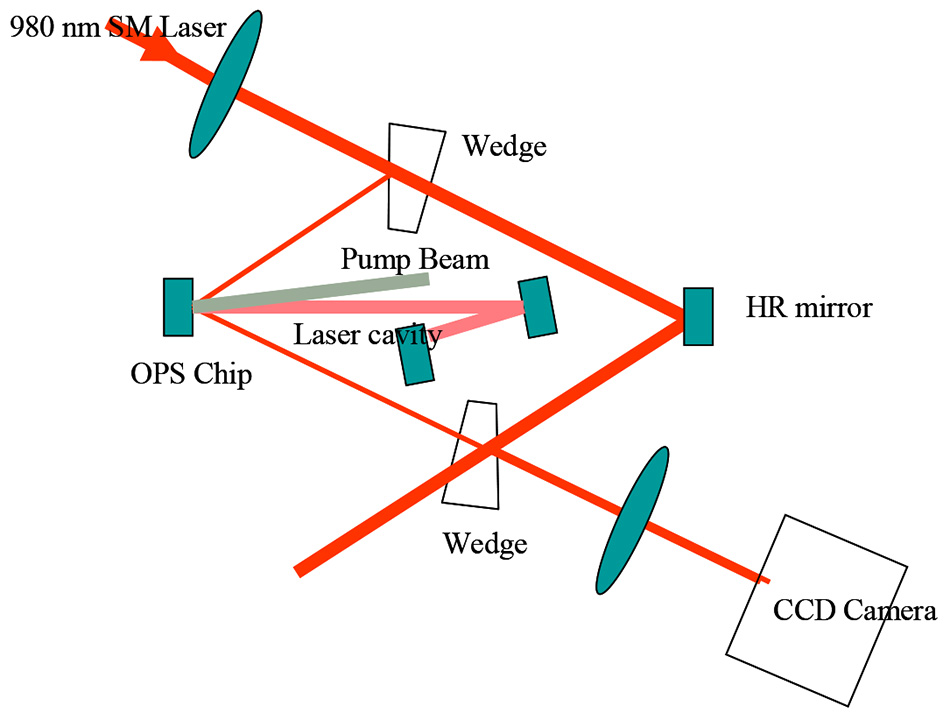
Figure 2: The optical performance of the OPSL gain chip was tested by incorporating it within a Mach-Zehnder interferometer based on a high-coherence single frequency 980 nm test laser.
In the tests, the pump laser in the OPSL was focused to a spot only 420 μm in diameter. The power of this pump laser was varied between zero and 9 watts. Even under this extreme thermal loading, the total wavefront distortion was barely detectable at ~ λ/40.
Actual Laser Performance Data
Of course, it is the real laser performance that matters under actual operation. To fully exploit this absence of thermal lensing requires a rugged monolithic cavity design where all other optics or optomechanics are insensitive to changes of the pump power. When thermal lensing occurs, the most pronounced changes to the output beam are observed in the beam divergence and beam diameter. These are also the most critical parameters for demanding applications such as laser-based imaging and pumping titanium sapphire lasers.
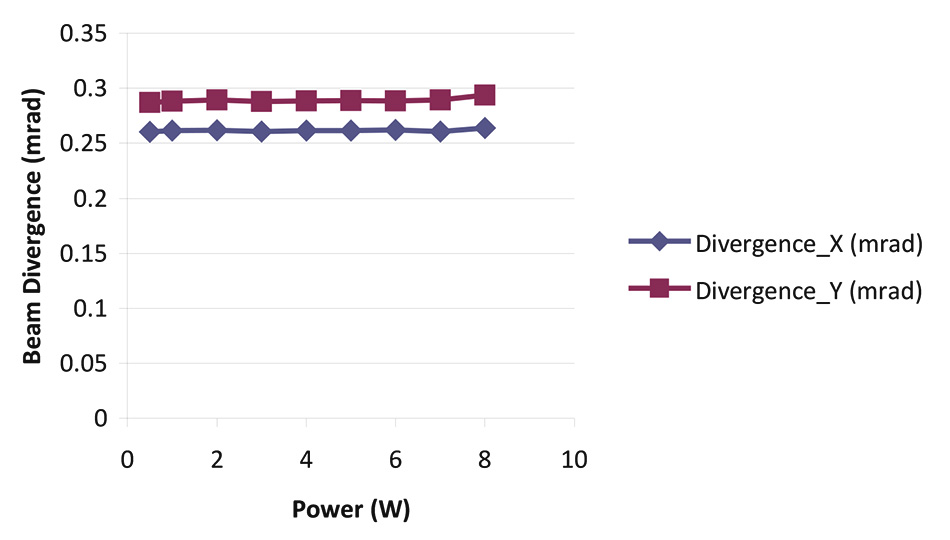
Figure 3: Varying the output power by over an order of magnitude in a Verdi series OPSL results in no meaningful change in output beam divergence.
Coherent engineers conducted comprehensive series of experiments to look directly for changes in these parameters, as a function of output power. Specifically, the 532 nm output of a 8 watt Verdi G laser was varied stepwise over an order-of-magnitude span, from a few hundred milliwatts to 8 watts. Even over this huge variation in output power, the beam diameter and beam divergence are both remarkably constant and stay well within specification as shown in the typical data sets in figures 3 and 4.
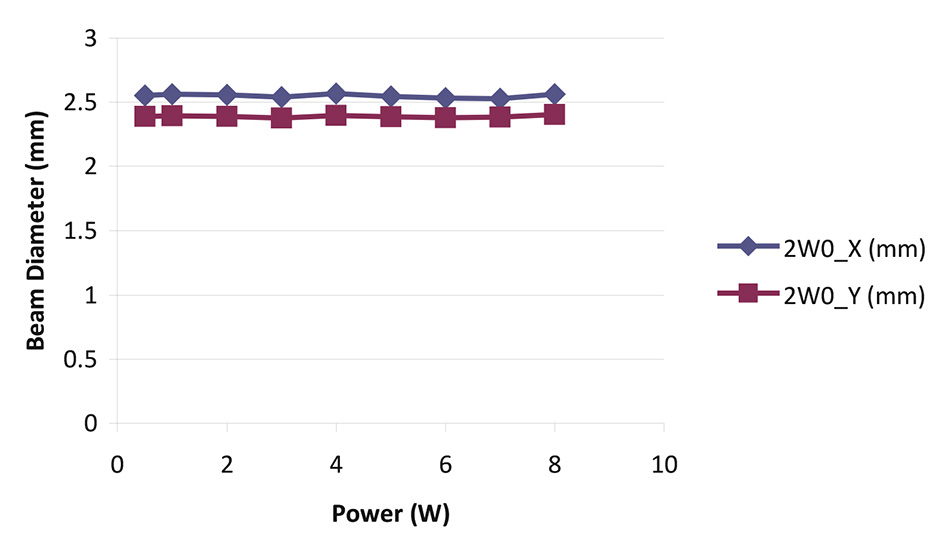
Figure 4: Varying the output power by over an order of magnitude in a Verdi series OPSL results in no meaningful change in output beam diameter.
Summary
The main technology choices for CW visible and near IR laser sources include DPSS lasers and OPSLs. The OPSL provides several unique advantages, one of which is the invariance of the beam parameters even when the pump (and output) power is varied by over an order of magnitude. Compared with other solid-state lasers, this advantage results in an easier system set-up and more convenient daily operations, ultimately delivering a better performance/cost ratio.