WHITE PAPER
Mephisto Lasers – Ultra-Low Noise and Narrow Linewidth
The Mephisto family of lasers delivers the combination of lowest noise and narrowest linewidth of any commercial continuous wave (CW) laser. The monolithic non-planar ring oscillator (NPRO) architecture, together with the active noise suppression, provides valuable optical tools to gravitational waves research, atom cooling and trapping, LIDAR, long-path interferometry, optical communications and other high-performance applications.
Introduction
When gravitational waves (GW) were detected for the first time in September 2015, it was not only the culmination of decades-long work, but also the beginning of an exciting new period in astronomy where space-time metrology data opened a completely new observation window – an achievement celebrated by the Nobel Prize in Physics in 2017. Few would argue that GW detection currently represents the most demanding application for narrow linewidth CW lasers. Researchers need the ability to observe modulations in space-time as small as 1 part in 1022. The preferred approach to detecting these tiny spatial-temporal shifts is a long-path interferometry using ultra-stable lasers. These interferometers (LIGO, GEO600, Virgo, KAGRA) all have kilometers-long arms angled at 90 degrees. To detect a GW, the system needs to be able to measure path difference equivalent to a trillionth of a wavelength – accuracy which is completely unprecedented in optical interferometry. To achieve the lowest possible noise, optical systems in all four previously mentioned GW observatories start with a Mephisto laser as a seed source. Its 1064 nm output is then amplified and further stabilized by a few orders of magnitude in both intensity and frequency to achieve the required parameters for the light that is injected into the interferometer arms [1].
Another application that is critically dependent on low noise lasers is trapping of cold atoms. Here, an amplified version of Mephisto (up to 55 W) is providing industry leading stability and linewidth combination and is used to form optical dipole traps for various species of cold atoms. An advanced version of this is the formation of optical lattices – an optically formed landscape of micro-traps where cold atoms are distributed. Optical lattices are seeing a wide use in research using cold atoms to study phase transitions, optical atomic clocks, diatomic molecules, and quantum simulators. Here, a laser’s optical stability in both intensity and phase is critical to ensuring lowest atom heating rates which, in turn, maximizes the experiment time. The above mentioned applications are only a few of many enabled by narrow linewidth, high stability, CW laser sources. LIDAR, optical frequency standards, squeezed light experiments, laser injection seeding, free-space optical communications, optical metrology, trapping of nanoparticles, and many others benefit from the parameters available from the Mephisto product line. In this white paper, we examine the technology and functionality behind the industry’s quietest laser product.
NPRO – Monolithic Stability
Since its invention in 1984/5 by Byer, Kane, and co-workers at Stanford University[2], the non-planar ring oscillator (NPRO) is still recognized as the lowest noise CW laser architecture available. One of the keys to low output noise is a stable laser cavity. Typical single-mode lasers incorporate a gain medium and various optics which reside in a resonant cavity formed by two or more mirrors supported by precision mechanical mounts. The NPRO uses a completely different approach to the laser cavity where a single crystal acts as both the gain medium and the laser cavity, which is defined by the crystal facets. Just as important, the NPRO acts as a unidirectional traveling wave ring oscillator. In linear laser cavities, the electric field vector of the highest gain mode (and all modes) follows a standing wave pattern along the cavity, causing “hole burning” – the gain is depleted (or undepleted) in a sinusoidal pattern along the cavity. Even though they have lower absolute gain, other longitudinal modes can still oscillate using the gain left undepleted by the strongest mode. Oscillations of undesired modes are eliminated, designing the laser to operate as a traveling wave resonator. This type of resonator relies on an optical diode to force oscillation in a single direction of propagation. An optical diode is a device whose forward transmission is much higher than its transmission in the reverse direction, resulting in a single longitudinal wave propagating along the forward direction only. Unlike a standing wave pattern, the traveling wave depletes all the available gain and prevents oscillations of other undesired modes. One of the clever elements of the NPRO’s design is that the crystal not only acts as the cavity, but also incorporates all the necessary elements to make an optical diode – all in a monolithic format.
The complete theory of unidirectional NPRO oscillation[3] is beyond the scope of this whitepaper. To summarize, the crystal is held in a strong magnetic field, which causes it to act as a Faraday rotator, rotating the polarization of the laser light passing along it by the same amount and direction independently on the beam direction of propagation (nonreciprocal rotation). In addition, the total internal reflections (TIR) at facets B and D (Fig. 1) impart a reciprocal rotation to the laser light inside the cavity. The net effect is that in one direction around the cavity the polarization rotations due to the magnetic field and the TIR add up, while in the opposite direction they cancel each other, resulting in two different polarization states for the two modes propagating in opposite directions. The output coupling coating on facet A can then be designed to slightly favor one polarization, resulting in unidirectional oscillations.
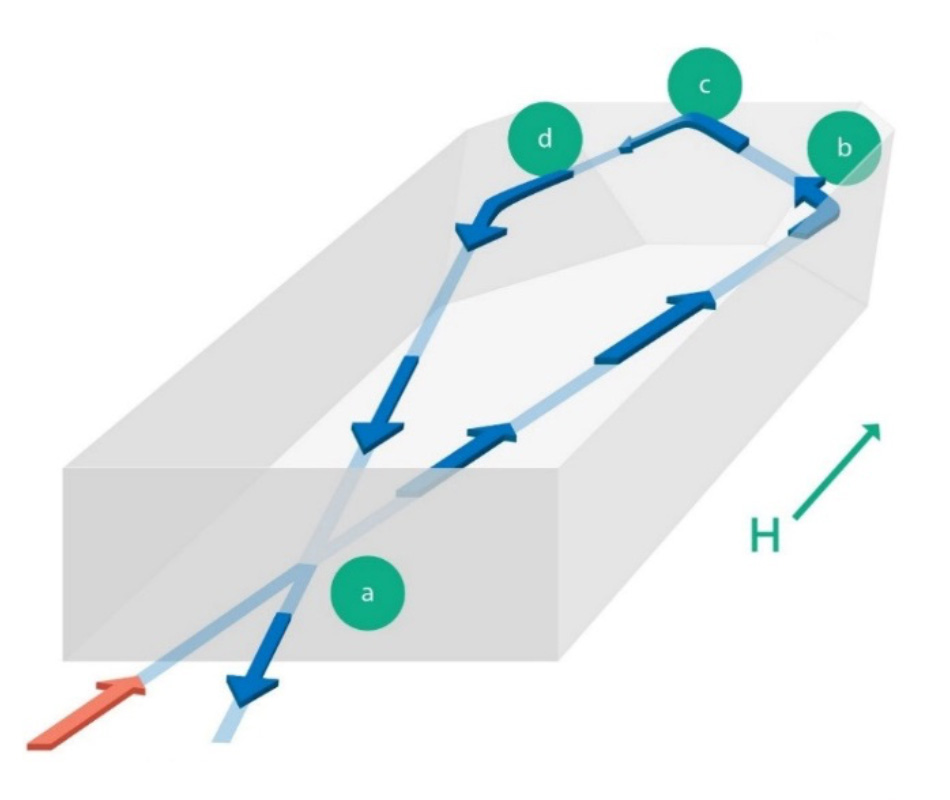
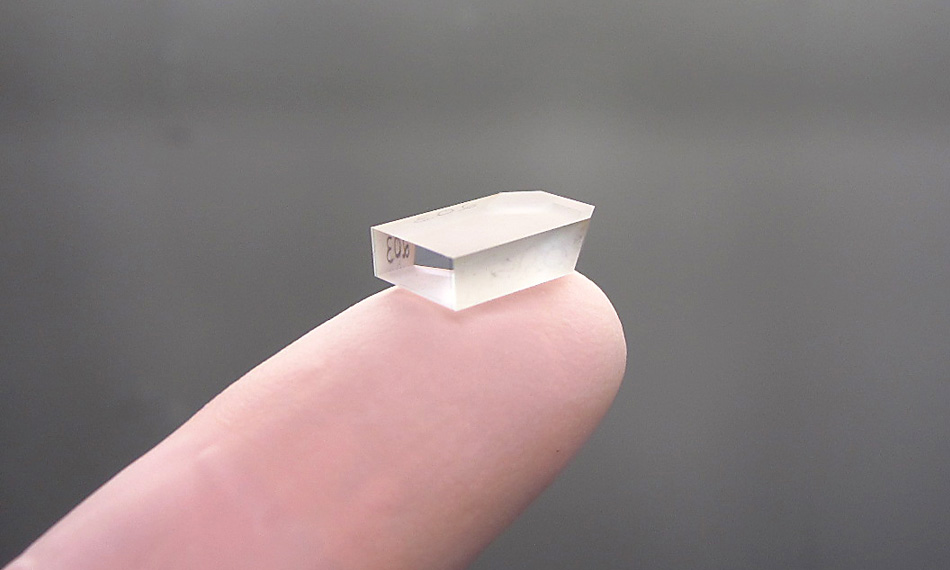
Size of the NPRO crystal which lives in the heart of Coherent’s Mephisto family of lasers.
Figure 1: A schematic illustration of the optical mode (blue arrows) inside the NPRO crystal (orange arrow represents pump light). NPRO gain crystal forms a monolithic laser cavity, where the facet angles ensure high reflectivity through total internal reflection (TIR). The facets are arranged so that polarization rotation (due to TIR), in combination with an externally applied magnetic field, preferentially supports unidirectional traveling wave laser operation.
Linewidth and Frequency Tuning
The frequency characteristics of the NPRO – i.e., linewidth – are superior to other laser types. Mephisto models provide ≤3 kHz linewidth straight from the laser, without any further stabilization. Even today, the latest fiber lasers and external cavity diode lasers struggle to reach the same linewidth performance in free-running operation.
Of course, a narrow linewidth resonator would be useful only for short term-applications unless frequency drifts can be actively controlled. In Mephisto, two different mechanisms are used to provide fast, fine control and slower, coarse control of the emission frequency. By extension, this control also provides the ability to tune the absolute frequency of Mephisto over the 30 GHz region.
Fast and fine control is achieved by use of a piezo electric transducer (PZT) element attached to one of the large non-optical facets of the crystal (i.e., one of the horizontal surfaces in Fig. 1). Compression (strain) of the crystal provided by the PZT element results in a change of the effective length (or refractive index) that leads to a shift in the frequency of the longitudinal modes. This high-speed loop can be operated with up to 100 kHz bandwidth and changes the mode frequency by 1 MHz/V.
Slower but larger frequency variations are addressed by varying the NPRO temperature (temperature tuning). Changes in the temperature of an Nd:YAG monolithic resonator shifts the frequency through two effects: the crystal thermal expansion coefficient and the temperature-dependent change in the refractive index. In addition to these effects, temperature changes can also cause very small shifts in the gain curve of Nd:YAG. Once this is factored in, the net effective change is approximately -3 GHz/K. Fig. 2 shows the measured temperature-based frequency control of Mephisto. Mode hops take place when the frequency of the oscillating mode (as determined by the resonator) shifts with respect to the center frequency of the gain-bandwidth by an amount similar to the resonator free spectral range (FSR). The full tuning range of Mephisto is ~30 GHz when the crystal temperature changes by 25°C. This value is lower than the 3 GHz/K value because of the mode hops. Special Mephisto versions can be provided with wider mode-hop free ranges (Extended Tuning Range option with 15 GHz of tuning between mode-hops). Options to shift the tuning range to lower frequencies (down to 281.565 THz) are also available for users working with specific spectral lines. Fig. 3 summarizes the overall tuning characteristics of Mephisto when both temperature and PZT tuning are exercised.
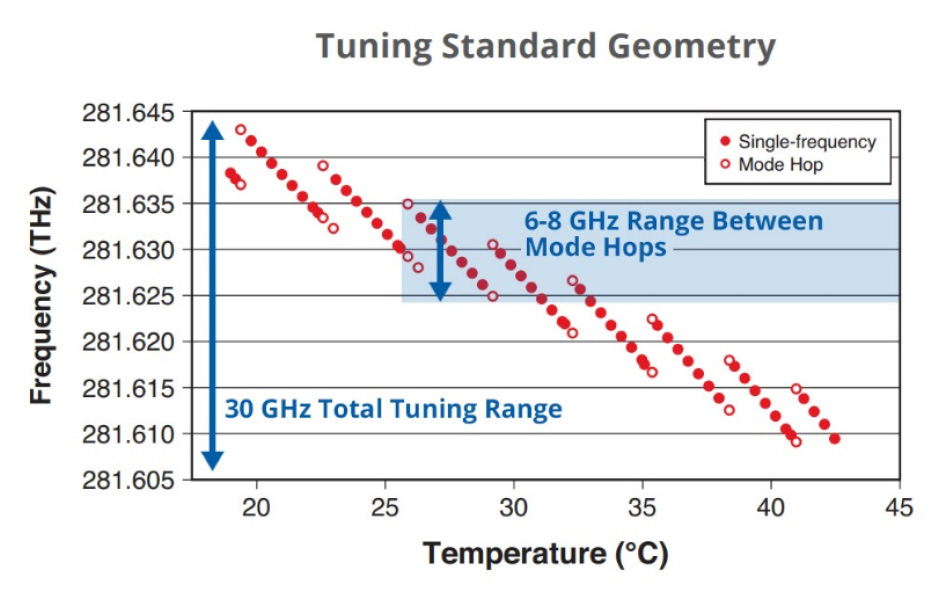
Figure 2: The Mephisto output frequency as a function of crystal temperature. Mode-hops and regions where two modes can simultaneously oscillate are also indicated.
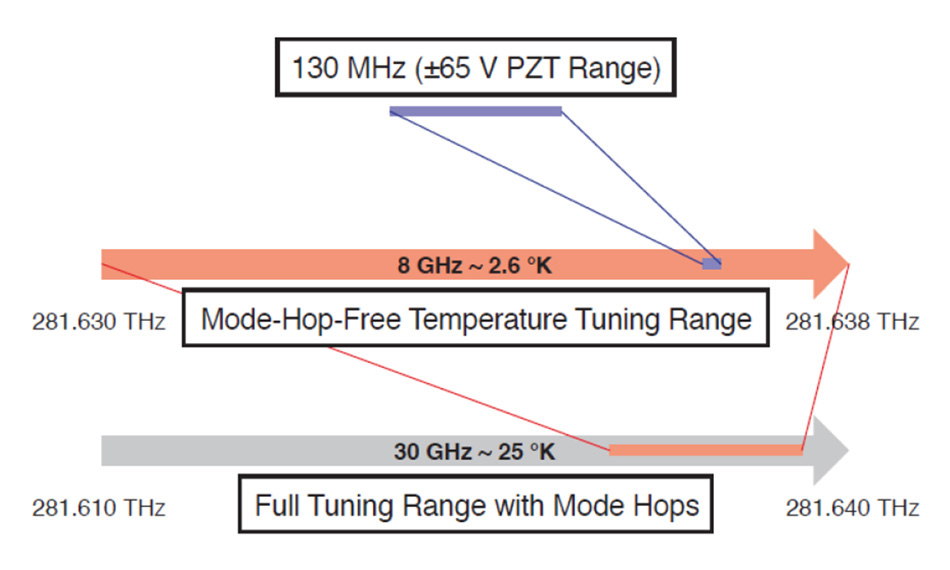
Figure 3: Mephisto frequency tuning ranges with PZT and temperature control.
Phase Noise and Frequency Locking
Phase Noise is the name for the error in the frequency linewidth of the laser. Not surprisingly, the monolithic Mephisto laser resonator yields a frequency noise performance superior to laser resonators made up of discreate cavity elements that are bound to vibrate or thermally drift with respect to each other. Depending on the intended application, this frequency jitter may be expressed in the form of a power spectral density (PSD) statistical distribution in units of Hz/√Hz.
When Mephisto frequency noise is expressed in this way, it follows a 1/f behavior. The frequency noise is about 104 Hz/√Hz at 1 Hz and goes down to 1 Hz/√Hz at 10 kHz (Fig. 4). Independent tests have previously shown that Mephisto provides the absolute lowest phase noise at a wide range of frequencies[4]. As a result of this unique performance, the Mephisto family of lasers is the optimum choice for applications where the lowest possible frequency noise is required.
The temperature and PZT tuning mechanisms can be used to smoothly tune the laser under external control and may also be used to lock the laser output to an external reference for those applications needing an absolute fixed frequency output. This locking also serves to lower the phase noise at low frequencies, compared to a free-running laser. The frequency doubled version of Mephisto (Prometheus laser providing both 1064 and 532 nm outputs) conveniently covers multiple molecular Iodine (I2) transition lines existing around 532 nm and can be used to lock its emission frequency to one of the hyperfine transitions of those lines. This makes it an ideal laser source for an absolute frequency reference and high-accuracy metrology. An example Allan deviation measurement of Iodine-locked Prometheus is shown in Fig. 6. Prometheus laser here is locked to the a10 component of the R(56)32-0 transition of the molecular iodine. The Allan deviation measurement, recorded over 20h period, indicates the relative stability of ~10-13 (modified Allan deviation averaged over 1s) down to 3·10-14 (averaged over 1000s). The Iodine locking work and measurements here were performed by TEM Messtechnik GmbH [6].
In the applications where absolute frequency is not necessary, and the user wants to stabilize the laser output at higher frequencies, Mephisto provides required functionality for locking the laser to the stabilized high-finesse cavities.
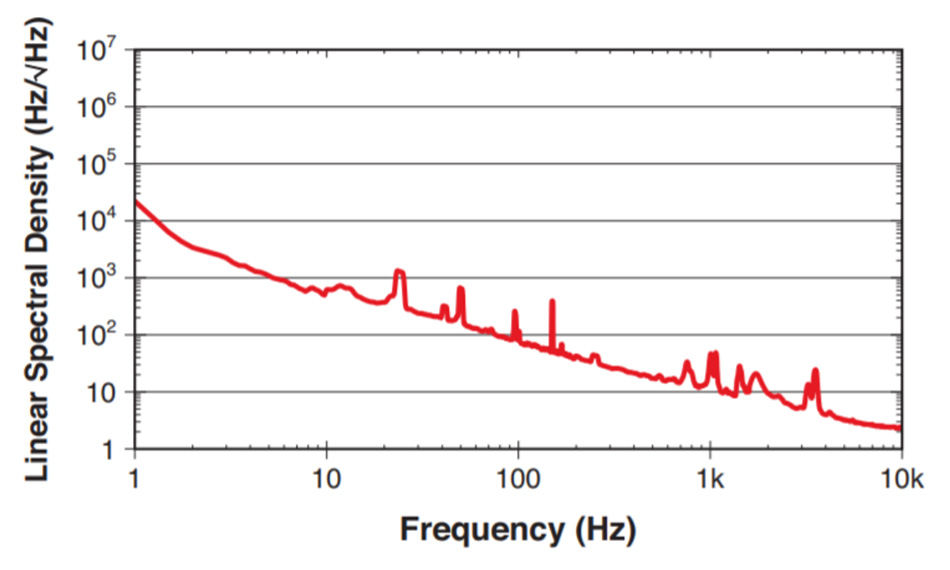
Figure 4: Mephisto Power Spectral Density performance follows 1/f behavior over a wide range of frequencies indicating the lowest phase noise.
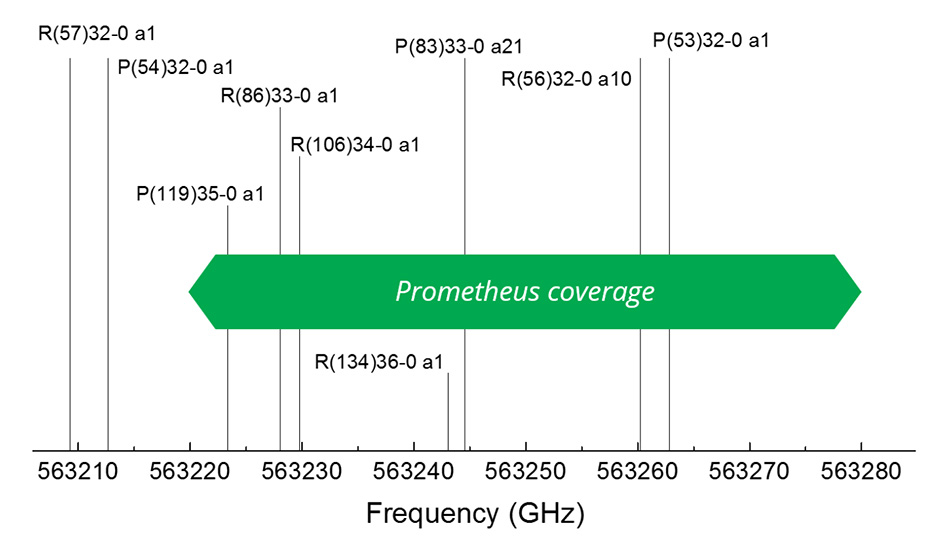
Figure 5: Molecular iodine (I2) transitions around 532 nm wavelength. The green band shows the region that can be covered by tuning Prometheus emission frequency. Adapted from[6].
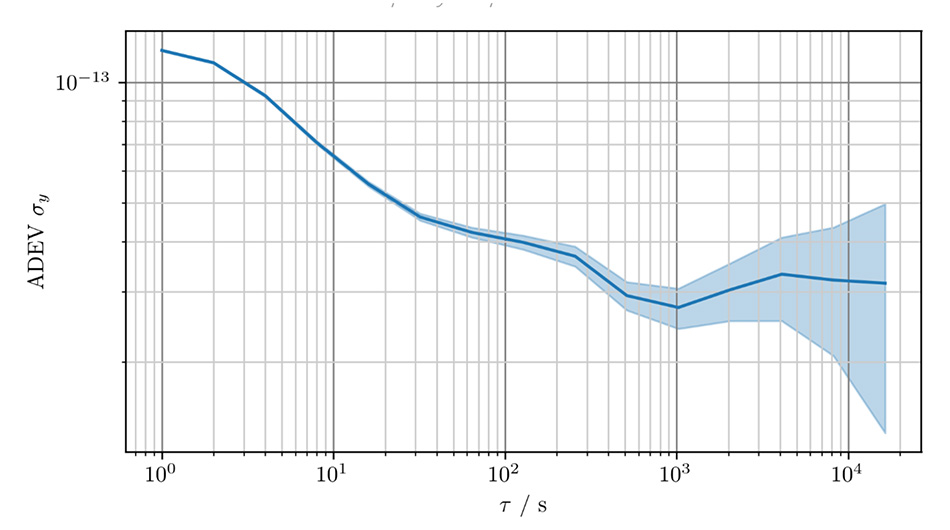
Figure 6: Modified Allan deviation measurement of the Iodine locked Prometheus laser frequency from its mean value over 20 hours period. The Iodine locking was performed by TEM Messtechnik GmbH [6].
Amplitude Noise and Noise Reduction
The Mephisto product line also features extremely low amplitude noise. As its name indicates, amplitude noise refers to any minor jitters in the output intensity. Amplitude noise is typically expressed as Relative Intensity Noise (RIN) which is the noise normalized to the average power level at which it is measured. In a diode-pumped solid state laser like Mephisto, the main sources of amplitude noise are typically relaxation oscillations produced by the residual pump diode noise.
Relaxation oscillations occur in any laser where the upper state lifetime is longer than the cavity damping time, i.e., the time for all the circulating power in the laser to decay (mostly through the output coupler losses) when the laser pump power is turned off. With diode-pumped lasers, when the laser diode pump power changes, even by a small amount, oscillation relaxations will take place producing a peak in the noise spectrum of the NPRO or any other solid-state laser – see Fig. 6. In Mephisto this peak is effectively eliminated by the use of a feature called Noise Eater. This is a built-in fast feedback loop with the driving signal provided by a photodiode within the laser head and acting on the pump laser diode current. Fig. 6 shows how effective this feature is at eliminating pump diode noise from the kHz to 2 MHz spectral region, as well as the relaxation oscillation peak. In addition to the Noise Eater, in order to support extremely low noise emission, Mephisto systems use specially designed low noise electronic controllers, largely based on analog design.
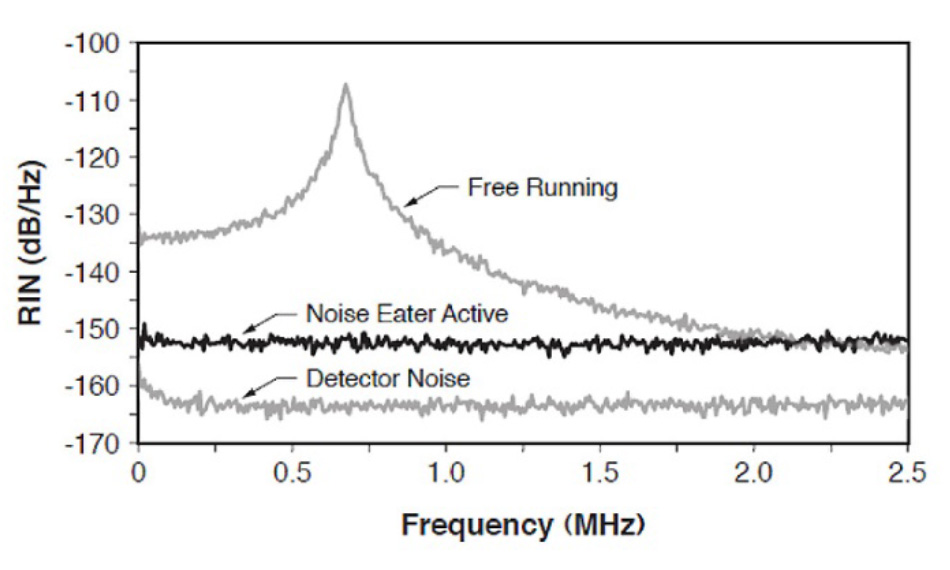
Figure 7: Mephisto amplitude noise, expressed as RIN (Relative Intensity Noise) is specified to be below -140 dB/Hz at frequencies above 10 kHz. The Noise Eater circuit is effective in eliminating most of the pump diode current noise as well as the noise peak due to relaxation oscillations.
Power Scaling and Wavelength Options
In general, as the pump power is scaled up, the NPRO will develop positive thermal lensing with two adverse consequences: the mode matching between pump diode and resonator mode is worsened and the resonator eventually becomes unstable. A detailed study of designing NPROs in the thermal lensing regime[7] shows that when the pump power is increased, the thermal lensing will lead to a reduction in the spot size of the mode. Ultimately this will result in a loss of mode matching between pump diode and the resonator fundamental mode, leading to multi-transverse mode oscillation and loss of the main functionality of the NPRO. From these considerations, it is clear that a high power NPRO is optimized for nominal output power rather than for low threshold or high efficiency when used at low power. Mephisto can achieve output power as high as 2 W, retaining the usual ultra-narrow linewidth, low noise and high frequency stability. If more power is desirable, the best path to achieve tens of watts while still retaining ultra-narrow linewidth is to use a Master Oscillator Power Amplifier (MOPA) approach, available with up to 55 W of power (Mephisto MOPA model).
Although NPRO crystals are most commonly available at the Nd:YAG fundamental wavelength of 1064 nm, specific applications can benefit from different wavelengths. For this reason, Mephisto lasers are also available at, frequency doubled version with green (532 nm) output (Prometheus model).
Rugged Design
The monolithic resonator design is also more rugged than typical lasers built from discrete components. Not only is the entire cavity enclosed in the bulk of the active medium, but the use of internal reflection from all surfaces except one minimizes the need to use optical coatings. Indeed, the only coated surface is the output facet, but here too, the actual intracavity reflection takes place inside the active medium. This means that the entire laser cavity is contamination-free by design and virtually impervious to damage and aging. This is in stark contrast to most other lasers where designers (and often end-users) have to worry about maintaining optical alignment and laser cavity cleanliness. Furthermore, the small size of the NPRO and pump diodes results in a small head size, making it straightforward to precisely stabilize the operating temperature and easy to integrate into OEM tools.
Summary
Mephisto lasers fully exploit the unique potential of the NPRO laser architecture. Their excellent laser beam parameters, unmatched combination of ultra-narrow-linewidth, frequency tuning, high power, and extremely low noise make the Mephisto family the laser of choice for demanding fields like atom cooling and trapping, optical communications, metrology, quantum optics, gravitational wave studies, and other applications benefiting from narrow linewidth, extremely stable laser sources.
References
[1] P. Kwee et al., Opt. Express 20, 10, pp. 10617-10634 (2012)
[2] T.J. Kane, R.L. Byer, Opt. Lett. 10, 65 (1985)
[3] A.C. Nilsson, E.K. Gustafson, R.L. Byer, IEEE J. Quantum Electron. QE25, 767 (1989)
[4] K. Numata et al., Proc. SPIE 10511, Solid State Lasers XXVII: Technology and Devices, 105111D (2018)
[5] J. Ye et al., IEEE T. Instrum. Meas. 48, 2, pp. 544 - 549 (1999)
[6] https://tem-messtechnik.de/en/
[7] I. Freitag, A. Tunnermann, H. Welling, Opt. Comm. 115, pp. 511-515 (1995)