WHITE PAPER
THz Research Benefits from Coherent Femtosecond Amplifier Power
The combination of high pulse energy and pulse repetition rate from a stable Coherent Elite Duo enables intense CEP-stable THz pulses which are used to interrogate high-field electron behavior in semiconductors.
Introduction
In the laboratory of Professor Rupert Huber at the University of Regensburg, a Coherent ultrafast amplifier model Legend Elite Duo is used to produce CEPstabilized THz pulses. These pulses are used to probe the behavior of electrons in GaSe samples under the influence of transient THz fields approaching 100 MV/cm. By electro-optical “stroboscopic” gating of the resultant signal with an 8 fs pulse in a GaSe detector, the data yields important information about Bloch oscillations as well as coherent and interfering conductive mechanisms only revealed at these high fields and short time intervals [1]. This information can be used in the nascent field of coherent electronics and potential coherent THz-rate electronic computing. As a side benefit, the signal radiated from the sample will be shown to take the form of an extended “ladder” of high-order harmonics uniquely covering from <0.1 THz in the far-infrared to 675 THz in the ultraviolet, all locked with complete phase stability, for use in photonic experiments. The high field amplitude and high-harmonic CEP-stable generation are enabled by the pulse energy and stability of the Legend Elite amplifier.
New Frontiers in Solid-State Electronics
The power and density of microelectronics continue their seemingly relentless increase in accordance with Moore’s Law. For many years, the main challenge in keeping pace with this overarching industry roadmap has been the fabrication of ever smaller structures, pushing microlithography far beyond the presumed diffraction limit. However, as gate lines and other features are shrunk down to only tens of nanometers, inherent material properties begin to present hurdles; witness the increasing use of low dielectrics, for example. New challenges will include other effects due to the extremely high electric fields and onset of coherent/quantum phenomenon resulting from shrinking physical dimensions. The continued advance of integrated electronics, including so-called coherent electronics, requires a deeper understanding of how electrons (and holes) behave under these extreme conditions.
For example, as electric fields can transiently exceed 1 MV/cm in the latest ICs, solid-state physicists want to know how fundamental charge transport mechanisms vary at fields of this magnitude and higher. This is because typical breakdown fields for many semiconductor materials are around 1 MV/cm and, if higher fields are applied, failure (even burning) will rapidly occur. One solution that enables even higher fields to be safely applied for a few femtoseconds is to use ultrafast THz pulses.
Why THz Pulses?
THz radiation is the portion of the electromagnetic spectrum sandwiched between the infrared and microwave regions. Because THz radiation with useful intensity is notoriously difficult to generate by conventional (e.g., blackbody) methods, it’s only with the recent development of laser-based methods and clever high-speed detection schemes that this radiation window has transitioned from a curiosity to an important tool in various scientific and commercial applications.
As described here, frequency mixing techniques with ultrafast lasers enable the production of coherent broadband THz pulses with energies as high 30 microjoules and pulse durations of a few tens of femtoseconds or less. These pulses are useful for studying semiconductors because when focused into a sample, they can generate local electric fields approaching 100 MV/cm. As the energy of a THz photon is two orders of magnitude or less than the typical semiconductor bandgap, THz high fields can serve as a precisely adjustable bias. Moreover, although the instantaneous field strength can be two orders of magnitude greater than the typical DC breakdown voltage for many semiconductors, the brief (femtosecond) duration of the pulses means that resultant high-field effects can be studied without the risk of material breakdown actually occurring.
Generating CEP-Stabilized Coherent THz Pulses
In 2008, the Huber group demonstrated a method of generating THz pulses where the phase between the electric field oscillations and the carrier envelope of the pulse is very stable and readily adjustable. The work outlined here is the first use of these CEP-stabilized THz pulses to study charge transport in a semiconductor, in this case, gallium selenide (GaSe). (Previous research studies by other groups had examined the effects of intense non-CEP stabilized THz pulses on semiconductors.) It was anticipated that the ability to tune the CEP delay could reveal hitherto hidden information on conduction pathways.
As shown in Figure 1, the heart of their setup is a Coherent Legend Elite Duo ultrafast amplifier, pumped by a pulsed Coherent Evolution laser. The amplifier is seeded by a titanium:sapphire Coherent Vitara laser oscillator that is pumped by a low-noise Coherent Verdi laser. The amplifier output beam is used to pump two tunable optical parametric amplifiers (OPAs). The signal wavelengths of these two OPAs are tuned to produce a terahertz frequency difference (e.g. 30 THz) between their outputs when combined in a suitable difference frequency generation (DFG) crystal. Use of the DFG technique ensures that the resulting pulses are “passively” CEP-stable, but without requiring a CEPstabilized pump laser.
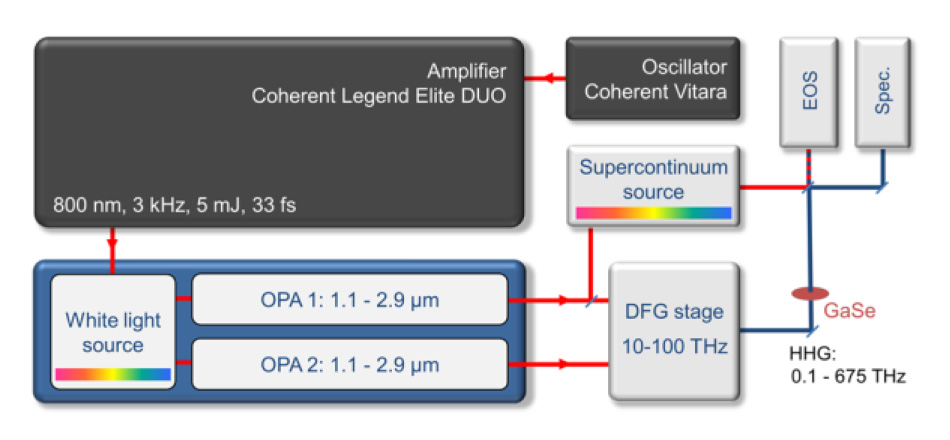
Figure 1. Schematic of the experimental system used in this THz research.
Compared to other methods of producing THz pulses, Huber’s method has several advantages. First the THz pulses are coherent, enabling high electric field generation when focused into a semiconductor or dielectric material. Also, the center wavelength of the THz pulse is readily tunable, simply by tuning the frequency (wavelength) difference between the two OPAs. Such pulses allow researchers the ability to study effects as a function of several parameters: electric field intensity, THz frequency, and CEP offset.
The Need for a Powerful Amplifier
The heart of the laser system used in this research is a titanium:sapphire amplifier, which was chosen primarily for its high pulse energy, high repetition rate (and hence high average power), superior beam quality, and cooling simplicity. We now examine why these characteristics are critically important and how the Legend Elite Duo uniquely delivers this combination of advantages.
According to Huber team member, Dr. Olaf Schubert, “High pulse energy is important for two reasons. First this leads to the highest THz pulse energy and hence the highest transient fields in the semiconductor sample. And just as important, this method of producing CEP stabilized THz pulses relies on using two OPAs that are completely phase correlated. The only straightforward way to achieve this correlation is to drive both OPAs from a single amplifier source which must provide both the amplifier driving power as well as a single white light seed pulse for both OPAs.” When the Huber THz setup was first assembled, the highest available pulse energy at the desired 3 kHz repetition rate was 5 millijoules from the Legend Elite Duo.*
In this type of study, a high repetition rate allows multiparameter experiments to be performed within shorter timeframes, in spite of all the non-linear interactions that these experiments entail. Legend Elite delivers high pulse energy even at 3 kHz, when pumped by the powerful Evolution -HE– a frequency-doubled Nd:YLF laser that provides up to 15 mJ/pulse at 3kHz.
Beam quality (i.e., low M2 ) and low noise are important because an OPA is a non-linear device. In fact its efficiency (and therefore output power) depends considerably on the beam quality of the pump laser. Additionally, THz generation and - more so - its higher harmonics are negatively affected by amplitude noise in the pump beam because they are non-linear processes where any input power fluctuation gets amplified. Better beam quality and noise can dramatically improve the SNR of the data and reduce data acquisition times.
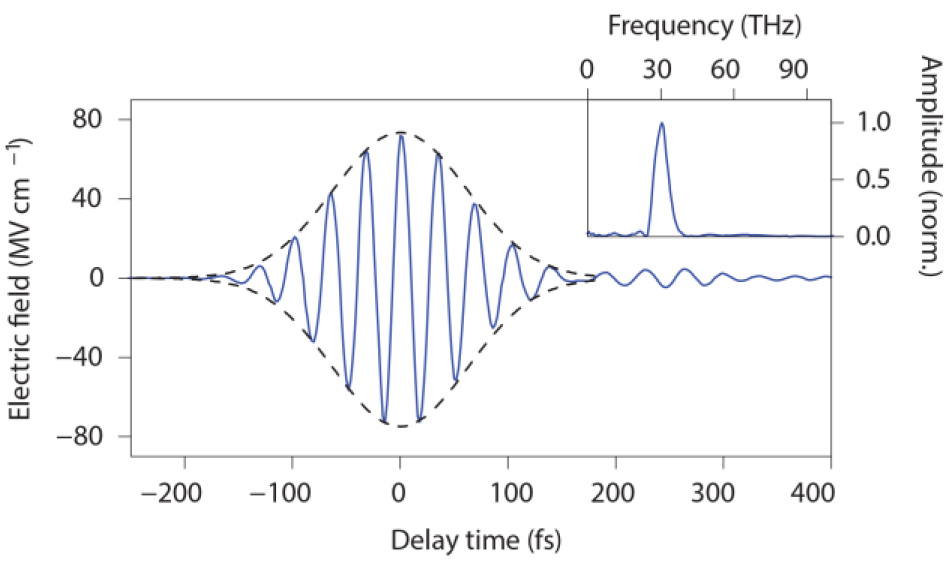
Figure 2. The waveform of the THz driving field (blue, solid curve) features a Gaussian envelope (black dashed curve) with an intensity full-width at half-maximum of 109 fs, which contains three optical cycles. The transient was recorded electro-optically in a GaSe sensor (thickness, 40 µm), with an 8 fs near-infrared gate pulse (center wavelength, 0.84 µm).
Coherent Legend amplifiers are designed to provide both short and long-term consistent high beam quality and noise, so that each experimental data-set is not only acquired rapidly but it is also very repeatable.
Electro-Optic Detection – THz Signal Sampling at Femtosecond Speed
As already noted, when focused into a semiconductor (GaSe) sample, the intense THz pulses create a 100 MV/cm electric field oscillating on a femtosecond time scale. The field excites electrons in the GaSe and their dynamics can be monitored with femtosecond resolution by “stroboscopically” detecting the THz radiation that is re-emitted as a result of this oscillating excitation.
These THz signal pulses are recorded using the Pockels effect. In a conventional Pockels cell, a high-voltage electric field is applied to a crystal such as KD*P. This causes the crystal to rotate the polarization of incoming optical radiation. The addition of a crosspolarizer results in an active optical switch. In the research described here, the THz field replaces the relatively slow electric field, inducing transient birefringence. Fast sensing of the THz field oscillations is performed by detecting polarization shifts in transmission of supercontinuum pulses generated by focusing part of the OPA output into a YAG crystal and then recompressing these spectrally broad pulses to an 8 fs pulsewidth - see figure 1. (In a series of earlier experiments, these pulses were generated from a fiber laser [2].)
In addition, the time-averaged signal is mapped in the frequency domain by means of a dispersive monochromator equipped with an InGaAs diode array and a silicon CCD. Together with the Pockels-detected data, this setup allows the emitted spectrum to be mapped from the terahertz regime through the far-infrared to visible spectrum
Electronics Implications – Bloch Oscillations and More.
Felix Bloch predicted 85 years ago that highly accelerated electrons in a periodic solid like these GaSe samples would undergo rapid oscillations because their effective wavelength would be on the same size scale as the crystal lattice [3]. (This is analogous to the well-known interference between photons and periodic structures at longer wavelengths). Bloch oscillations in natural solids are nearly impossible to observe however, because of the very fast scattering of the electrons [4]. By using femtosecond THz pulses, the excitation timescale becomes comparable or faster than the scattering process and the oscillating electrons emit detectable electro-magnetic radiation throughout the frequency range 0.1 – 675 THz.
In technical terms, – as the THz excitation pulse rapidly switches the external electric field, the electrons undergo transitions between valence and conduction bands. These would not be possible in linear optical absorption because of the low photon energies. Moreover, the details of the resultant emission are quite sensitive to change in the CEP offset of the exciting pulse.
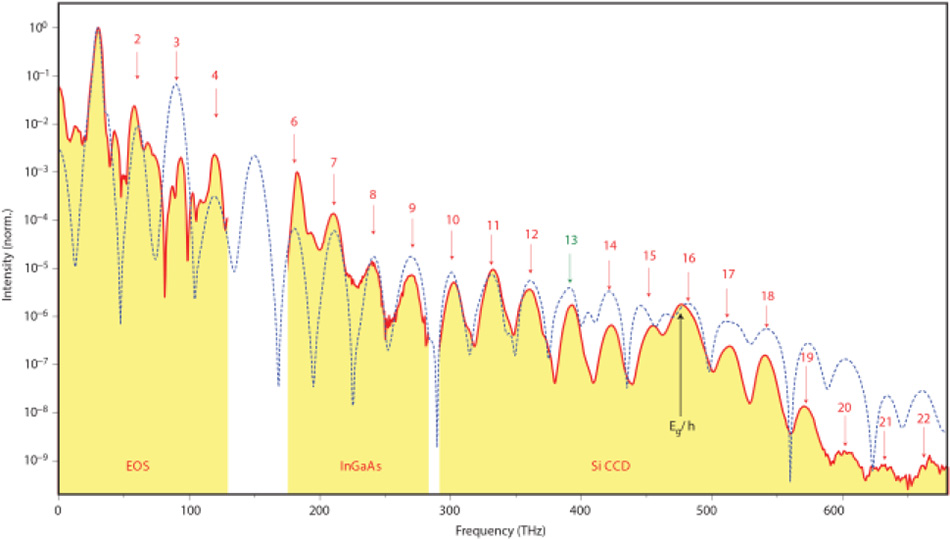
Figure 3. The oscillating excitation of electrons in the GaSe sample results in emission of an extended ladder of harmonics from 0.1 THz up to 675 THz.
The groups of Stephan W. Koch and Mackillo Kira at University of Marburg, in collaboration with Torsten Meier at University of Paderborn, have successfully analyzed this dependency, by developing a full quantum many-body theory, going far beyond Bloch’s seminal prediction [5]. In simple terms, they showed that three different valence bands and two conduction bands are involved. This complex situation provides multiple pathways for excitation between the valence and conduction bands (see figure 4). They show that the observed CEP dependence is a result of interference between the different pathways.
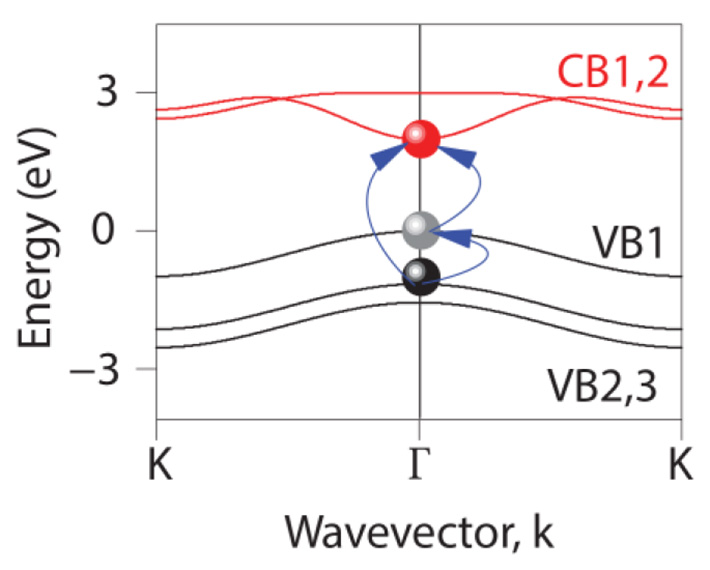
Figure 4. Electrons can move between five different bands in GaSe – three valence bands and two conduction bands - providing multiple excitation pathways.
In simplified terms, these unique data reveal previously hidden quantum electronic phenomena relevant for future semiconductor devices at teraflop clock rates. Specifically, they provide a first window into a novel regime of high-field charge transport on timescales of a single cycle of light.
Photonics Implications – Phase-Locked High Harmonics
From a photonic viewpoint, the nature of the radiation emitted by the GaSe is equally interesting and potentially of widespread importance and utility. First, this radiation covers an incredibly broad range of harmonics and hence frequencies. This stretches all the way from a fundamental frequency at <0.1 THz right through the visible to the 22nd harmonic at 675 THz, before the signal strength naturally decays and also surpasses the wavelength range of the photodetectors.
Two aspects of this comb of frequencies make it a useful and unique tool in its own right to perform other science on the femtosecond timescale. First, it is an extremely long ladder of harmonics and second, all the harmonics are coherent and precisely locked in phase, even though they span such a huge range of the electromagnetic spectrum. Huber’s group confirmed this CEP stability by performing a standard f-2f interferometry comparison by frequency doubling e.g. the 6th harmonic and detecting the interference between this and the 12th harmonic. This revealed CEP stability at the microradian level over a complete ten-minute measurement interval.
Summary
In order to support the development of integrated semiconductor circuits at ever higher densities and faster speeds, new frontiers in semiconductor physics must be explored. Energetic and stable femtosecond laser amplifiers provide unique tools to investigate highfield, high-speed solid-state physics by pumping highly non-linear processes like THz region harmonic generation. In addition to revealing new information about coherent electronic effects under these conditions, an important side benefit is the generation of an extended ladder of phase-stabilized harmonics of femtosecond duration across the entire THz through visible range of the electromagnetic spectrum. This ladder in turn will likely prove to be a useful tool for cutting-edge photonic experiments.