What is a Powell Lens?
Powell lenses are optics used to generate a uniform-intensity laser line. They employ a unique cylindrical aspheric surface shape to accomplish this. Powell lenses are used in applications as diverse as machine vision and flow cytometry.
Most lasers emit circular or elliptically shaped beams (in cross-section). The intensity profile across the beam is typically Gaussian or very nearly so. This Gaussian intensity distribution is beneficial for many applications. But there are also instances in which a uniform intensity distribution (often referred to as a “flat-top”) is more desirable.
There are several ways to convert a Gaussian beam into a uniform intensity distribution (in both one and two dimensions). The most powerful and flexible way is based on the Powell lens. Let’s look at why flat-top beams are sometimes needed, how the Powell lens works, and what benefits it offers over other technologies.
Gaussian Beams – The Good and the Bad
The drawing illustrates a circular Gaussian beam. This beam is much more intense in the center than at the edges. A Gaussian beam is the natural result of the physics underlying most lasers, which is why it is so common.
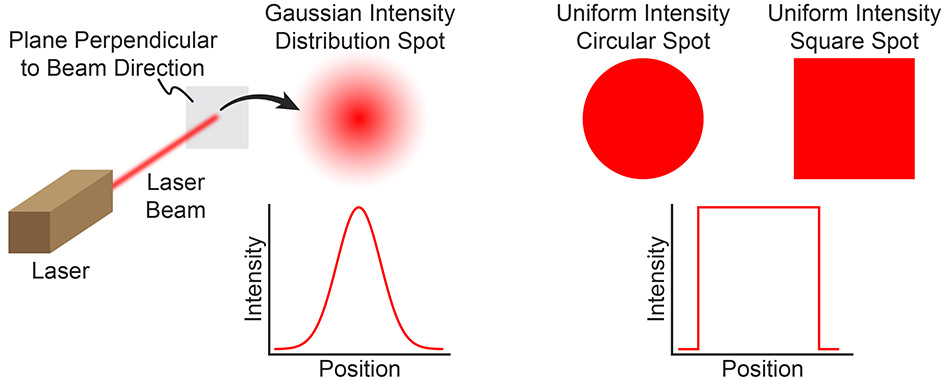
Most lasers naturally produce a round cross-section beam with a Gaussian intensity distribution – which is much brighter in the center than at the edges. A uniform intensity beam, both circular and square, is shown for comparison.
The Gaussian profile beam often turns out to be advantageous over a uniform intensity beam (either round or square) for several reasons. A key one is that a Gaussian beam can be focused to a smaller spot than a uniform circular beam of the same diameter. This comes in very handy in many applications. For example, in most materials processing uses, a smaller focused beam improves the ability to produce minute features, In many laser-based microscopy techniques, smaller focused spot size increases image resolution.
But there are some cases in which the opposite is true, especially when the laser is being formed into a line beam (a beam longer in length than width). Line beams are used in many illumination tasks. Uniform intensity is desirable here because evenly illuminating a scene or object simplifies subsequent image processing and increases image contrast and resolution.
Transforming Gaussian Beams
A unique property of Gaussian beams is that when they’re focused, expanded, or otherwise reshaped using traditional optics, they maintain their Gaussian intensity profile. It’s actually quite difficult to get rid of it.
The most simple and direct way to transform a Gaussian beam into one having a uniform intensity distribution is to pass the beam through an aperture which simply blocks all but the central, and most uniform, portion of the beam. There are two disadvantages to this approach. First, a very large fraction of the laser power is discarded – as much as 75%. Second, the resulting beam still isn’t all that uniform.
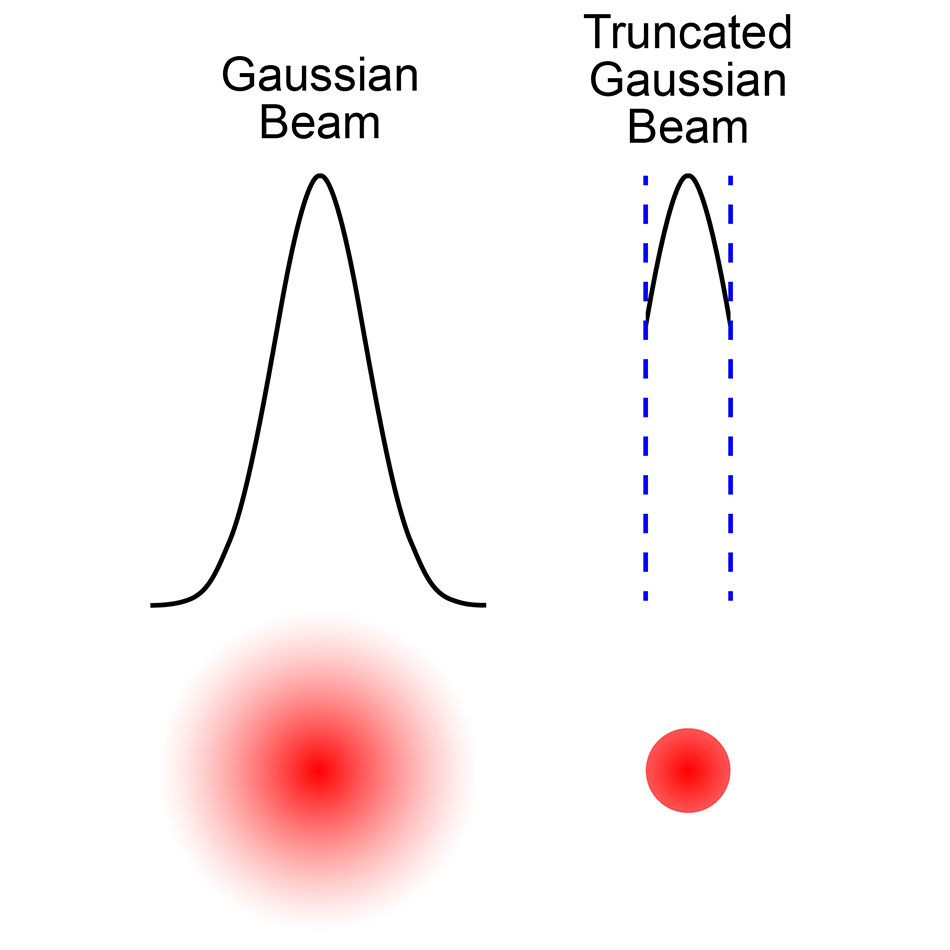
The simplest way to transform a Gaussian beam to a uniform intensity profile is to just select out the center portion of the beam and discard the rest. But this approach yields the poorest results.
Transforming a Gaussian beam to flat-top distribution without throwing out a lot of light is more difficult. But it can be done with both diffractive and refractive techniques.
Diffractive optics work by creating interference between various diffracted orders to spatially redistribute the light in a laser beam. This can produce virtually any arbitrary intensity profile, including nearly flat-top, as well as a wide variety of patterns.
There are two big negatives of diffractive optics for making uniform line beams. First, they’re not very efficient. They lose a fair amount of light into unwanted diffraction orders. Second, they are typically very wavelength-sensitive. This is particularly problematic when using them with diode lasers.
Lenslet arrays are a purely refractive approach. These are optics containing multiple lenses, each much smaller than the input beam. The output pattern produced by each lenslet overlaps in the far field, creating the desired uniform intensity distribution.
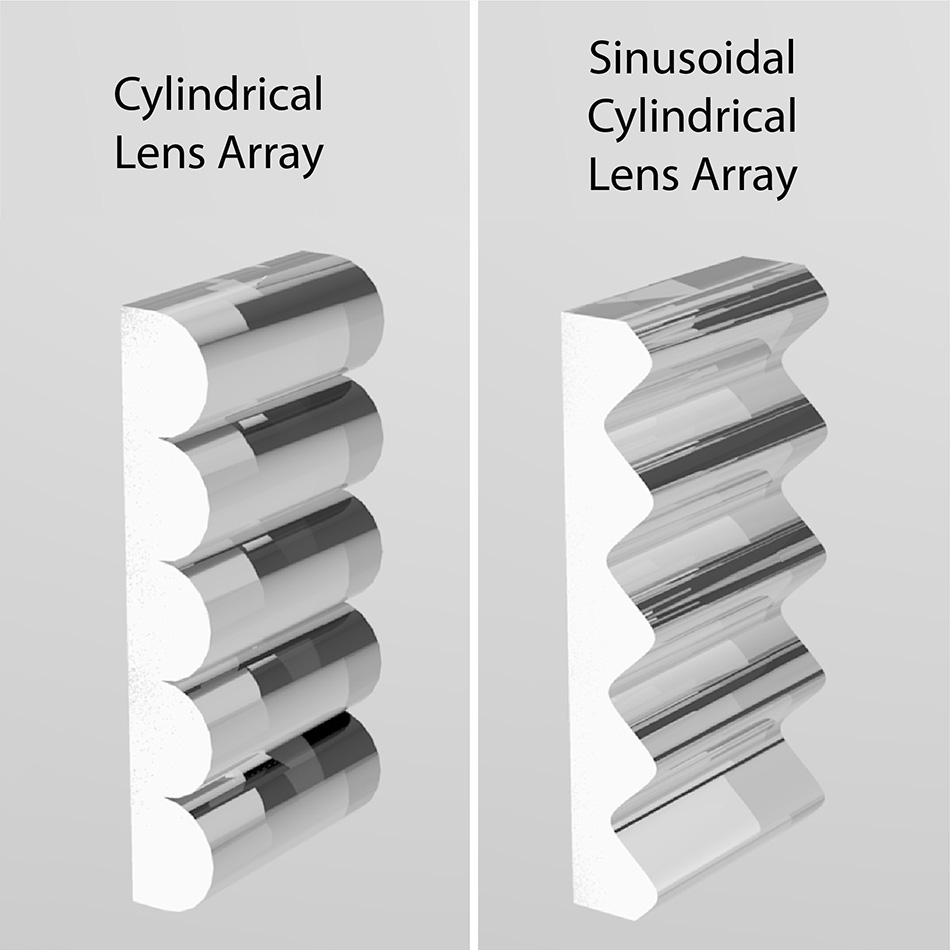
Two configurations of cylindrical lenslet arrays.
It’s very difficult to achieve a high degree of uniformity in the final beam with lenslet arrays. There is usually a substantial amount of high-frequency ripple in the intensity. Additionally, lenslet arrays also require specialized tooling to produce, limiting their usefulness to high-volume applications.
The Powell Lens
Powell lenses are another type of refractive optic that overcomes the limitations of both diffractive optics and lenslet arrays. The Powell lens is an aspheric cylindrical lens shaped specifically to efficiently transform a Gaussian input beam into a diverging beam with a uniform intensity distribution. Because a Powell lens is a type of cylindrical lens, it only homogenizes the beam in one dimension.
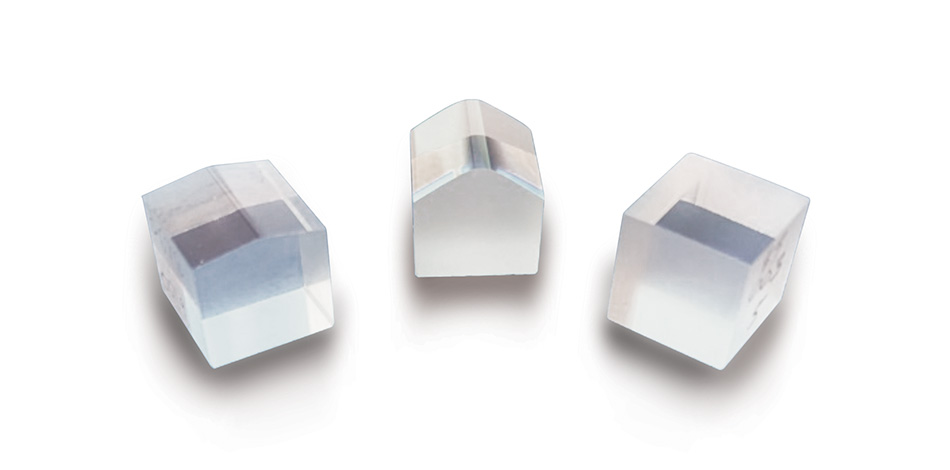
Powell lenses.
The figure illustrates the shape of the Powell Lens surface and compares its operation with that of a traditional cylindrical lens. The Powell Lens redirects light from the center to the edge of the beam in order to eliminate the central “hot spot.” The cylindrical lens also causes the beam to fan out in one dimension but preserves its Gaussian profile. So, it produces a line beam that is much brighter in the center than at the edges.
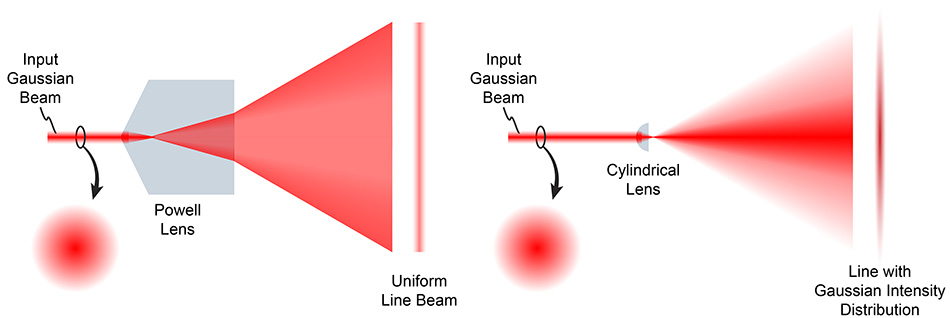
The Powell lens (left) is compared to a traditional cylindrical lens (right). Both optics transform a round Gaussian profile laser beam into a diverging fan of light which makes a line on any surface on which it is projected. The Powell lens shifts light from the center to the edge of the beam to produce a uniform intensity line, while the cylindrical lens maintains the Gaussian profile of the beam, so its line is much brighter in the center.
The Powell lens delivers superior results over diffractive optics in almost every aspect of performance. Most importantly, the Powell Lens is more efficient (loses less light), and produces a steep-edged pattern, with little light outside the desired region.
This Powell lens is also fairly insensitive to input wavelength. This makes it usable with diode lasers, since it is unaffected by unit-to-unit variations in wavelength, as well as the inherent bandwidth and wavelength temperature dependence of these sources. The result is that an overall intensity uniformity of ±5% over the entire pattern can be routinely achieved in production beam homogenizers without having to wavelength select or bin diode lasers.
Powell lenses aren’t perfect, though. Each is designed for a specific input beam diameter and won’t produce optimal results with larger or smaller beams. They are also sensitive to alignment (in the axis perpendicular to the cylindrical surface). Misalignment reduces the intensity uniformity of the projected line.
Understanding Powell Lens Specifications
Fabricating the cylindrical asphere on a Powell lens with high precision is challenging, and the quality of Powell lenses varies between manufacturers. This means the performance of a real-world Powell lens may depart significantly from its design values. This makes it important to know how to read published specifications.
The primary specifications for a Powell lens are its operating wavelength, intended input beam diameter (which is defined to be at the 1/e² intensity points, since the input beam is Gaussian), and output beam fan angle. These are shown schematically in the drawing. Since the output beam is intended to be uniform, and not Gaussian, the fan angle is measured at the point at which the power has dropped to 80% of its peak value (rather than the 1/e² power point).
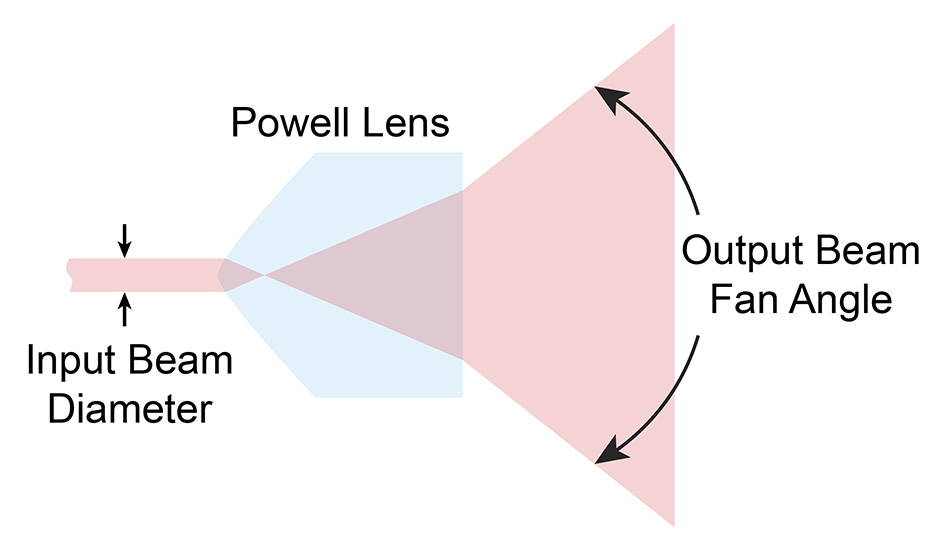
Primary nominal specifications for a Powell lens are the input beam diameter and output beam fan angle.
Typically, the most important performance parameter for a Powell lens is its intensity uniformity. Manufacturing variations and tolerance effects can produce intensity variations (particularly at the beam edges), non-flat top profiles, periodic structure, and scatter.
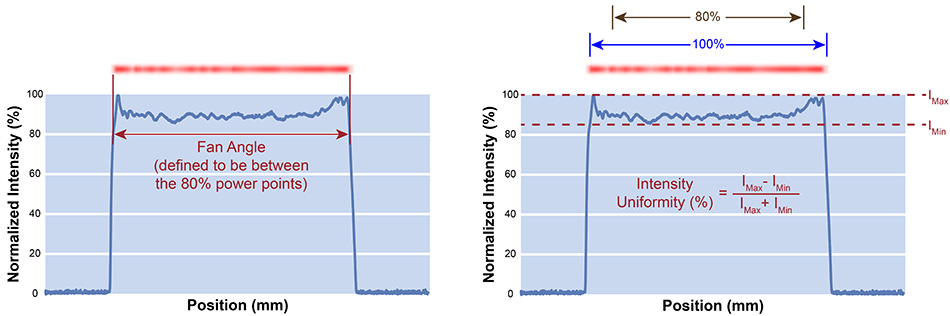
Power lens fan angle is usually measured from the points at which the intensity has dropped to 80% of its peak value. Intensity uniformity is specified differently by various manufacturers.
Most manufacturers define line uniformity using the formula given in the preceding drawing. However, they typically only apply this specification to the central 80% of the line (also indicated in the drawing). But excluding the edges of the beam creates an unrealistic picture of actual performance, because this is where non-uniformities are typically most pronounced.
In contrast, Coherent applies the intensity uniformity specification over 100% of the line length. The same applies to our specifications for line straightness and contained power (the ratio of power contained in the line between the 80% and the 1/e² peak power points). This is a much more stringent and difficult-to-meet specification. The result is the Coherent Powell Lens deliver better measurement accuracy, signal-to-noise ratio, and unit-to-unit consistency.