WHITE PAPER
Combustion Analysis with CARS – It Really is Rocket Science
Overview
The space launch industry is taking off at an accelerated rate – literally and figuratively. With the increased number of launches comes a recognition that all these rocket engines need to burn as cleanly as possible, with minimized negative impact on the atmosphere. Dr. Alexis Bohlin is a leading researcher applying a variety of Coherent Anti-Stokes Raman Scattering (CARS) methods – all enabled by the unique Astrella ultrafast amplifier – to analyze diverse combustion systems. His proven techniques and insights will soon be applied to the quest for cleaner rocket propulsion designs at the Kiruna Space Campus of Luleå University of Technology (Sweden) where Bohlin has recently accepted an appointment as Senior Researcher and an opportunity to work closely with the modern rocket industry establishment at the Esrange Space Center.
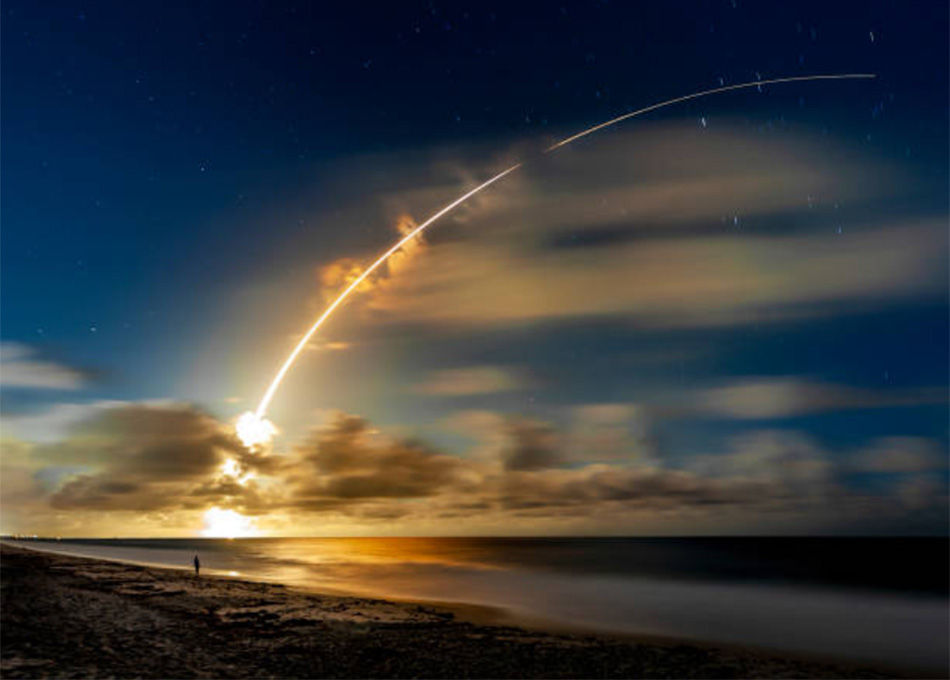
Figure 1: Engineers are targeting more efficient propulsion systems to minimize the atmospheric impact of a rapidly growing commercial space launch industry.
CARS – Measuring Species Number Densities and Temperatures
Dr. Bohlin explains his research focus on Coherent Anti-Stokes Raman Spectroscopy (CARS) for combustion analysis, “From automobiles, to furnaces, to rocket engines, combustion engineers want to improve system efficiency and reduce the amount of harmful emissions. Every combustion source is a complex chemical reactor and successful manipulation of combustion conditions depends on first knowing as many details as possible. Laser diagnostics have the unique ability to quantify scalars in the reacting flow, without perturbing the measurement region of interest, and the useful information can be provided with excellent spatial and temporal resolution. My research is focused on the use of CARS to map effective temperature and species number densities (i.e., concentration), for molecules including N2, O2, H2, CH4, C3H8, CO2, H2O and so on, with as great a precision and accuracy as possible.”
"Every combustion source is a complex chemical reactor and successful manipulation of combustion conditions depends on first knowing as many details as possible."
- Dr. Alexis Bohlin - Senior Researcher, Space Propulsion Laboratory
Luleå University of Technology, Kiruna, Sweden
The basic concepts of CARS are shown in figure 2. The molecular sample is irradiated with three laser frequencies (wavelengths), called the pump, the Stokes, and the probe, that interact with the sample through a third order non-linear optical mechanism to generate a fourth wavelength, the CARS, which is sent out as a “laser-like” coherent signal. When the difference between the two frequencies, the pump and the Stokes, corresponds to a difference between two energy levels in the probed molecules, the signal strength is resonantly enhanced by multiple orders of magnitude. Through such resonant enhancement a spectrum of the sample’s energy levels can be efficiently obtained using a single-laser shot where the intensity of each spectral peak depends both on the species number density, and on the populations of each species’ internal energy levels. In this way CARS tells you how much of each chemical species is present in the gaseous sample while the shape of the spectrum tells you the local Boltzmann temperature by consisting of pure-rotational or rotational-vibrational transitions.
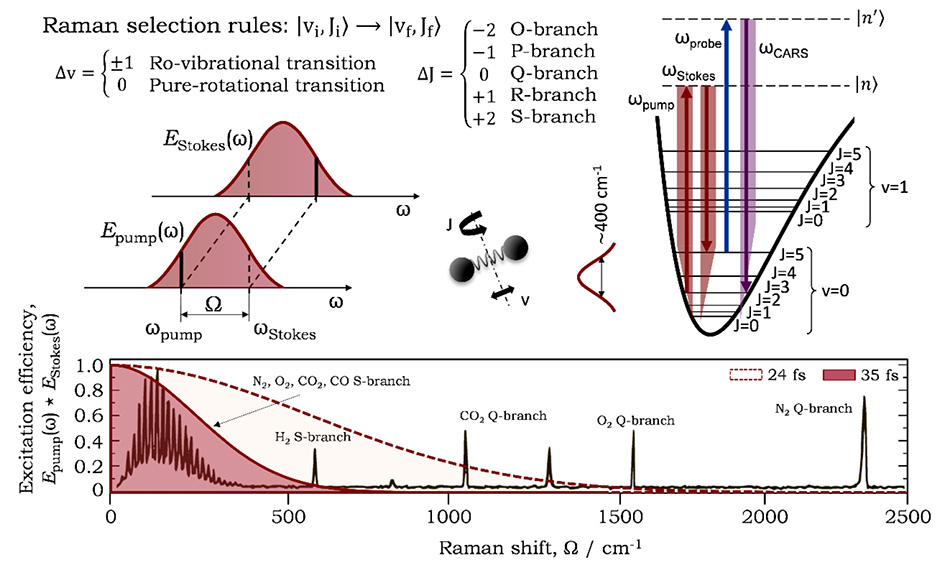
Figure 2: The excitation efficiency for driving the CARS transitions is dependent on the femtosecond laser pulse duration / bandwidth. The shorter the pulse, the more transitions can be coherently excited. Figure courtesy Alexis Bohlin.
CARS with a Single Ultrafast Amplifier
Since the goal is to map as many transitions as possible via impulsive excitation, CARS today is usually performed with ultrafast lasers pulses. Bohlin explains, “We want a femtosecond duration pulse for the pump/Stokes beam as this has a broad spectral bandwidth covering many of the energy levels of interest simultaneously. The short pulse duration < 50 fs can be considered impulsive for most diatomic- and triatomic species, and it provides the most efficient form of exciting the molecules. We then need a tunable narrowband probe pulse, i.e., a picosecond duration pulse, so that the CARS spectrum – the signal in figure 2 - consists of well-resolved spectral features of our various molecular targets. And if you can get both pulses from the same laser source, these become automatically synchronized at the measurement location, which greatly simplifies the setup, it also reduces the signal beating between closely spaced transitions shot-to-shot, and it increases signal-to-noise.”
In his laboratory at Delft University of Technology (the Netherlands) Bohlin and his students built a CARS analysis setup based on a Coherent Astrella “one-box” femtosecond amplifier configured for 35 fs output pulses. The high (several millijoules) pulse energy of this amplifier enables them to split the output and use part of it directly as the broadband pump/Stokes pulse. The other part is then used to generate a picosecond duration pulse with a device called a Second Harmonic Bandwidth Compressor (SHBC). After the SHBC a home-built pulse shaper is used to tune the pulse duration in the regime of ~3-15 picoseconds.
With this as their basic CARS engine, Bohlin and colleagues have successfully performed a variety of different studies on combustion flames and systems, perfecting techniques such as pure-rotational CARS with in-situ monitoring of the excitation efficiency, developing space-time CARS, cascaded CARS, and using cutting-edge concepts including self-phase modulation to generate and expand the necessary laser wavelengths “behind the windows”, in the combustion chamber itself.
Emphasizing Simplicity, Precision and Accuracy
Perhaps the most important aspects of Bohlin’s CARS system, are its stability and relative simplicity compared to more traditional approaches. And over the last few years Bohlin has made innovative improvements toward those ends. He states, “We wanted a universally applicable method that we could take to the combustion site if necessary, rather than a lab-bound method which could only be used on smaller engines that could be brought into the lab. You obviously can’t bring even a test rocket engine into a typical research lab. The use of a single laser source with the simplicity and stability of Astrella has played a huge role in making a portable system a reality.”
In terms of CARS performance, he has been purposefully targeting measurements with the world’s best precision and accuracy. Bohlin explains, “The history of laser spectroscopy shows that measuring experimental parameters with greater detail doesn’t just put decimal points on some numbers. Rather it often reveals important new science.” As an example of his approach, just by using the 400 nm output of the SHBC pumped by Astrella rather than the more usual 532 nm CARS laser wavelength, he has reduced the point spread function in his CARS imaging, from 40 microns to 20 microns.
He is now re-establishing a “canonical burner” for H2 flames where the standardized performance details will be quantified with unprecedented accuracy and precision. By looking at things that are fundamental to hydrogen flame propagation in greater fidelity, such as steep thermal gradients and diffusion problems, he is checking old theories and assumptions based on measurements made in the 1990’s.
Several of the recent advances in CARS combustion analysis deployed by Bohlin merit closer examination.
"We wanted a universally applicable method that we could take to the combustion site if necessary, rather than a lab-bound method which could only be used on smaller engines that could be brought into the lab."
Thermometry with Simultaneous Space and Time Resolution
In 2020 his group published a paper [1] demonstrating simultaneous – correlated – space (1D) and time (1D) resolution obtained with a single regenerative amplifier. Most analytical Raman measurements traditionally have focused on vibrational transitions, often with rotational-vibrational resolution from smaller molecules. Instead Bohlin has used pure-rotational CARS as this provides an optimum data format for precise thermometry and imaging. In this study, the team performed 1 kHz cinematographic 1D-CARS gas-phase thermometry across an unstable premixed methane/air flame-front, achieved with a single-shot precision <1% and accuracy <3%, 1.4 mm field of view, and an excellent <20 µm line-spread function. Here the signal generation plane was relayed by a wide-field coherent imaging spectrometer onto the detector plane. This was refreshed at the same repetition rate as the Astrella amplifier system that produced the naturally synchronized femtosecond and picosecond pulses as described above – see figure 3.
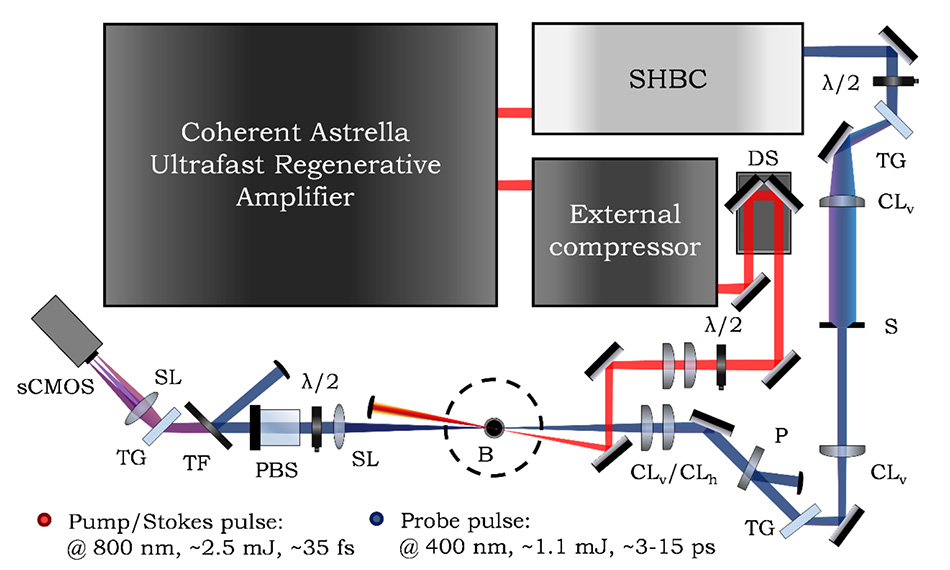
Figure 3: A single Astrella amplifier is used to generate synchronized femtosecond pump/Stokes and picosecond probe beams for CARS thermometry. Figure courtesy Alexis Bohlin.
CARS with In-Situ Referencing of the Excitation Efficiency
Another important development from the Bohlin group is their innovative polarization-sensitive coherent imaging spectrometer, which allows for the simultaneous recording of resonant and non-resonant CARS signals on the same detector frame [2]. He explains, “This detection scheme can be used to acquire in-situ information about the impulsive excitation efficiency, which has been an unknown in all previous femtosecond spectroscopies. Although the complexity level is quite high for this new protocol to become widely employed, it provides a unique pathway to go beyond the currently established accuracy and precision levels of CARS, which is already considered to be the benchmark for gas-phase diagnostics. With this innovation we have the chance to become truly calibration free, and I see a clear prospect of this method to push the performance of scalar determination to the ultimate dream-limit of delivering ±1% precision and ±1% accuracy.”
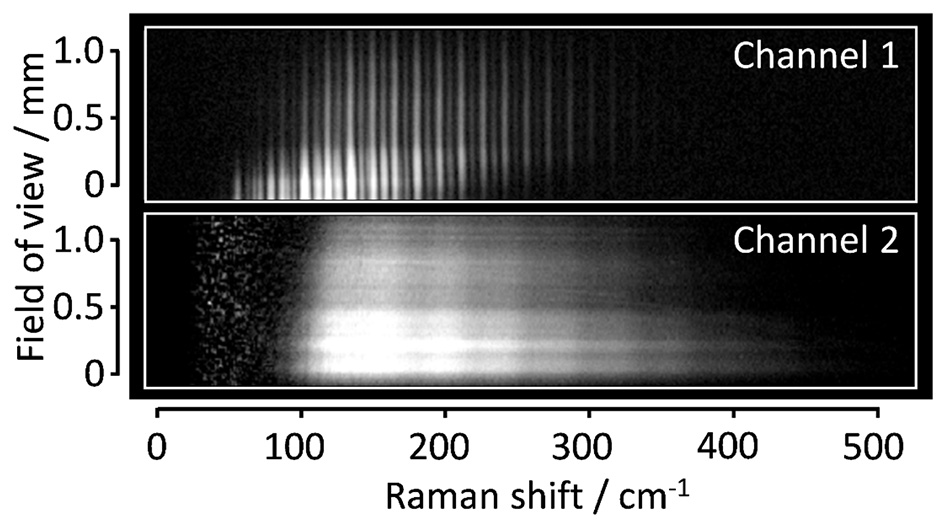
Figure 4: A resonant and a non-resonant CARS signal are generated and detected simultaneously, on the basis of a single-laser-shot with the Astrella. The resonant CARS signal (in channel 1) carries information about the temperature and species concentration in the sample, and the non-resonant CARS signal (channel 2) maps the effective bandwidth of the femtosecond laser pulse recorded in-situ. The information is needed to push the accuracy and precision of CARS scalar determination below the dream-limit of <1% [2].
Cascaded CARS – Highly Sensitive to Number Density
Cascaded CARS takes the whole CARS concept one step further so that the stimulated CARS signal itself becomes the probe pulse generating a higher order CARS signal from the sample! With multi-species targets as found in combustion systems, this might be expected to produce spectra of unusable complexity. However, Bohlin’s group have demonstrated that the spectra are actually perfectly amenable to computer analysis [3]. But why use a technique guaranteed to generate signals that are both weak and rather complex to analyze? He explains, “The signal strength is incredibly sensitive to number density – it scales as (number density)4. So it really supports our mission to push the sensitivity of CARS species measurements. It can for instance be used as a powerful laser diagnostic to quantify even tiny fluctuations in bulk mixture composition under well-mixed conditions. The ability to precisely determine, understand, and control a combustible mixture preparation process has always been very important for designers of efficient, clean-burning reaction engines.” He notes that the signal strength is also very sensitive to laser intensity, so the high stability of Astrella is critically important here in terms of pulse energy as well as the temporal and spectral profiles of the pulse.
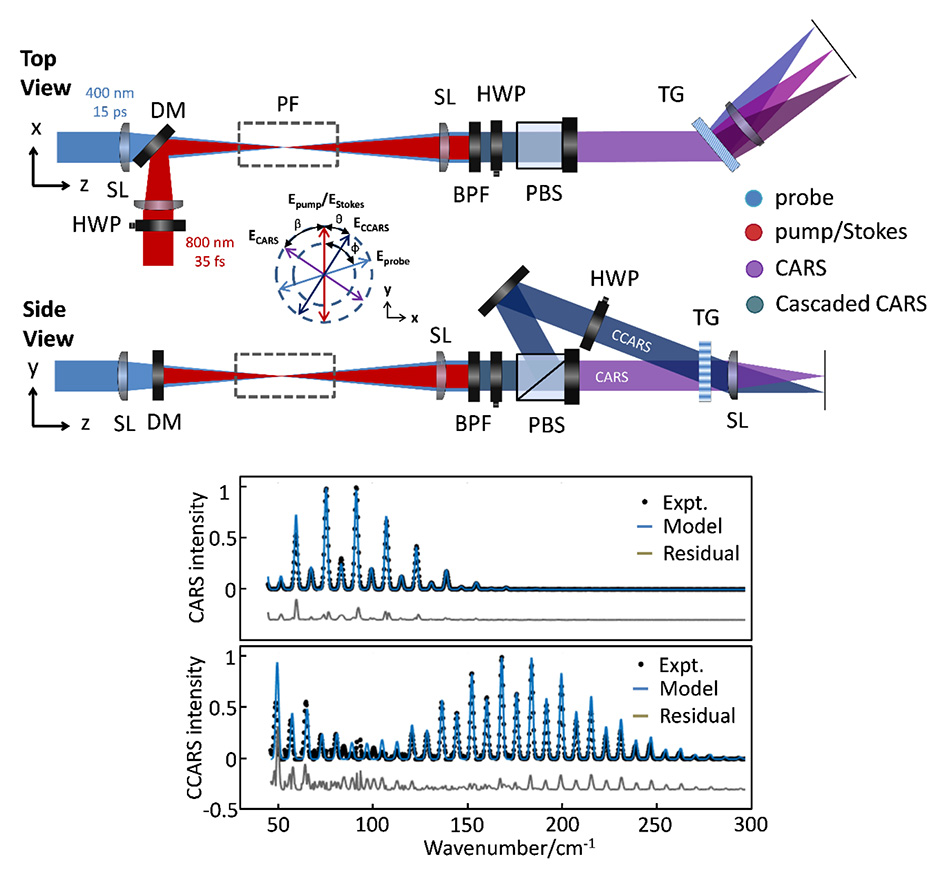
Figure 5: The simultaneously generated cascaded CARS and CARS signals are split and detected on the same frame with a polarization-sensitive coherent imaging spectrometer. In cascaded CARS, the stability of Astrella is critical to ensuring stable impulsive efficiency across the entire rotational spectral band(s) of interest [3].
Ultra-Broadband CARS with Self-Compressed Pulses
While the thermometry and cascaded CARS methods target one or two species in a flame, Bohlin’s group has also demonstrated a method capable of simultaneously monitoring all the major species relevant to combustion, e.g., O2, H2, CH4, and CO2. This uses an established method of generating ultra-broadband femtosecond pulses in-situ. They exploit this method to perform what Bohlin calls “soft compression” of the Astrella output: from 35 fs to about 24 fs, by means of femtosecond laser-induced filamentation actually generated in the flame [4]. This gives them access to the “fingerprint region” 1200-1600 cm-1 spanning rotational-vibrational signature bands of all the chemical species just listed. This compression technique creates a transform limited output at the trailing edge of the filament and the CARS probe volume is then formed ~4 mm after the filament intersecting the fs- and ps- beams. Bohlin explains, “Generating the ultra-broadband pulses in-situ is an incredible advantage that simplifies the optical setup, by avoiding the need to use additional pulse compression devices and chirp compensation optics. For example, the monitoring access window on a rocket propulsion unit can be 2.5 cm thick glass. The dispersion of femtosecond pulses due to such a thick window would be near-impossible to otherwise accommodate. Our ability to use a highly controllable filamentation in-situ generation method instead is yet another testament to the incredible stability of the Astrella output.”
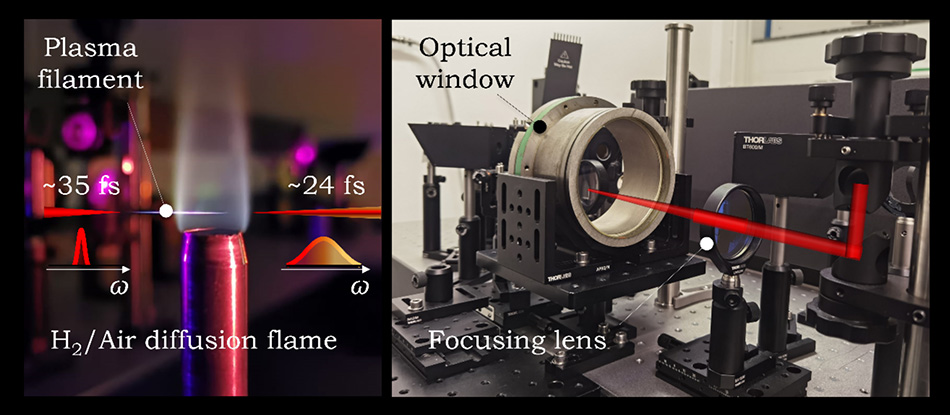
Figure 5: In ultra-broadband CARS, pulses spanning the entire 1200-1600 cm-1 region are created inside the flame, enabling monitoring through even thick glass windows with no dispersion compensation issues. Image courtesy of Alexis Bohlin.
"Our ability to use a highly controllable filamentation in-situ generation method instead is yet another testament to the incredible stability of the Astrella output."
Research that is Really Taking-off.
To summarize, the research group led by Alexis Bohlin at Delft University of Technology have demonstrated the power and versatility of CARS imaging and spectroscopy to map thermal gradients and number densities in a variety of flames and combustion sources. Their studies are connected by a common theme of increasing precision and accuracy,and their experimental setups all use Astrella as the common critical laser element. Bohlin will now apply these methods to analyze rocket propulsion systems at the Kiruna Space Campus, LTU and the Esrange Space Center in Sweden.
References
1. L. Castellanos, F. Mazza, D. Kliukin, A. Bohlin, Pure-rotational 1D-CARS spatiotemporal thermometry obtained with a single regenerative amplifier system, Opt. Lett. 45, 4662-4665 (2020). [Editor’s Pick]
2. F. Mazza, L. Castellanos, D. Kliukin, A. Bohlin, Coherent Raman imaging thermometry with in-situ referencing of the impulsive excitation efficiency, Proc. Combust. Inst. 38, 1895-1904 (2020).
3. D. Kliukin, F. Mazza, L. Castellanos, A. Bohlin, Cascaded coherent anti-Stokes Raman scattering for high-sensitivity number density determination in the gas-phase, J. Raman Spectroscopy; 1-9 (2021). [special issue].
4. F. Mazza, N. Giffioen, L. Castellanos, D. Kliukin, A. Bohlin, High-temperature rotational-vibrational O2-CO2 coherent Raman spectroscopy with ultrabroadband femtosecond laser excitation generated in-situ, accepted to Combustion and Flame in 2021.