WHITE PAPER
OPSL Advantages Whitepaper Series #3:
No Mode Noise (“Green Noise”)
Overview
The optically pumped semiconductor laser (OPSL) is a unique, patented technology that provides lower intrinsic noise than other continuous wave (CW) solid state lasers having UV or visible output, and is the only way to achieve true-CW output at 355 nm in a simple, cost-effective platform. The reason is the absence of mode noise due to the near-zero upper state lifetime of the OPSL gain medium.
OPSL Advantages White Papers in this series:
#1. Wavelength flexibility
#2. Invariant beam properties
#3. No mode noise ("green noise")
#4. Superior reliability - huge installed base
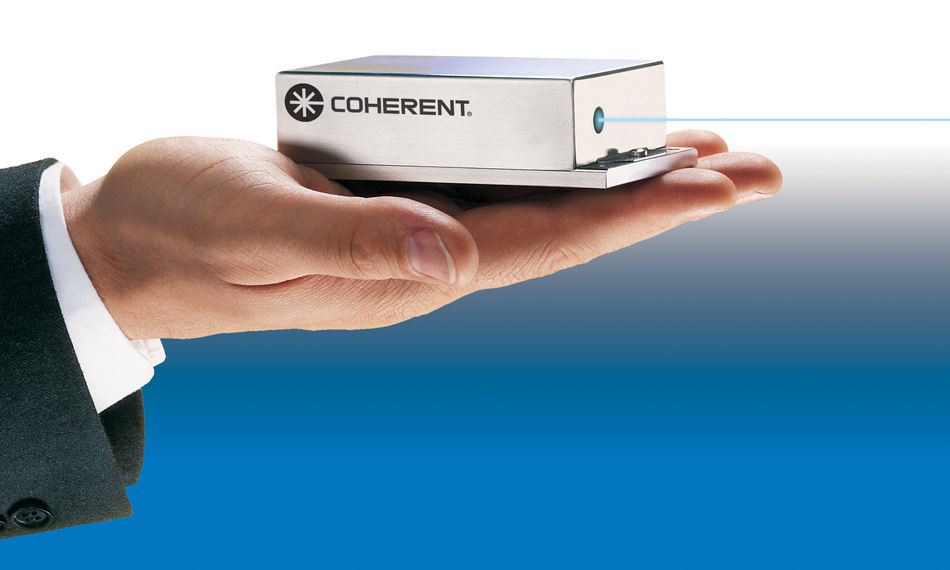
Eliminating the Performance/Cost Trade-off
Demanding visible and ultraviolet applications for CW lasers (e.g., pumping CEP-stabilized laser systems, Brillouin scattering, and semiconductor wafer inspection) need a high quality stable output beam with low amplitude noise. Diode pumped solid state (DPSS) lasers can produce the requisite beam quality, albeit only at a fixed output power1, but their noise performance is often limited by a problem called mode noise or “green noise.” Addressing this noise adds laser complexity. In DPSS visible lasers, there is thus a trade-off between performance (noise) and cost (complexity). This critical noise mechanism is completely absent in visible OPSLs which can thus offer lower noise at a reduced cost. It also enables OPSLs to generate noise free ultraviolet output (e.g., at 355 nm) in a simple format. This is a major reason that OPSLs dominate the use of ultraviolet wavelengths in flow cytometry.
1 See whitepaper #2 in this series.
Chaotic Mode Behavior
The output of continuous wave lasers based on a macroscopic cavity is highly dependent on the cavity configuration. This is true of OPSLs, legacy DPSS lasers and most CW gas (ion) lasers. With cavity lengths measured in tens of millimeters or even tens of centimeters, these CW lasers can support multiple longitudinal cavity modes. Usually in such lasers, the intra-cavity beam intensity is divided between multiple longitudinal modes, each with a slightly different frequency (see Figure 1).
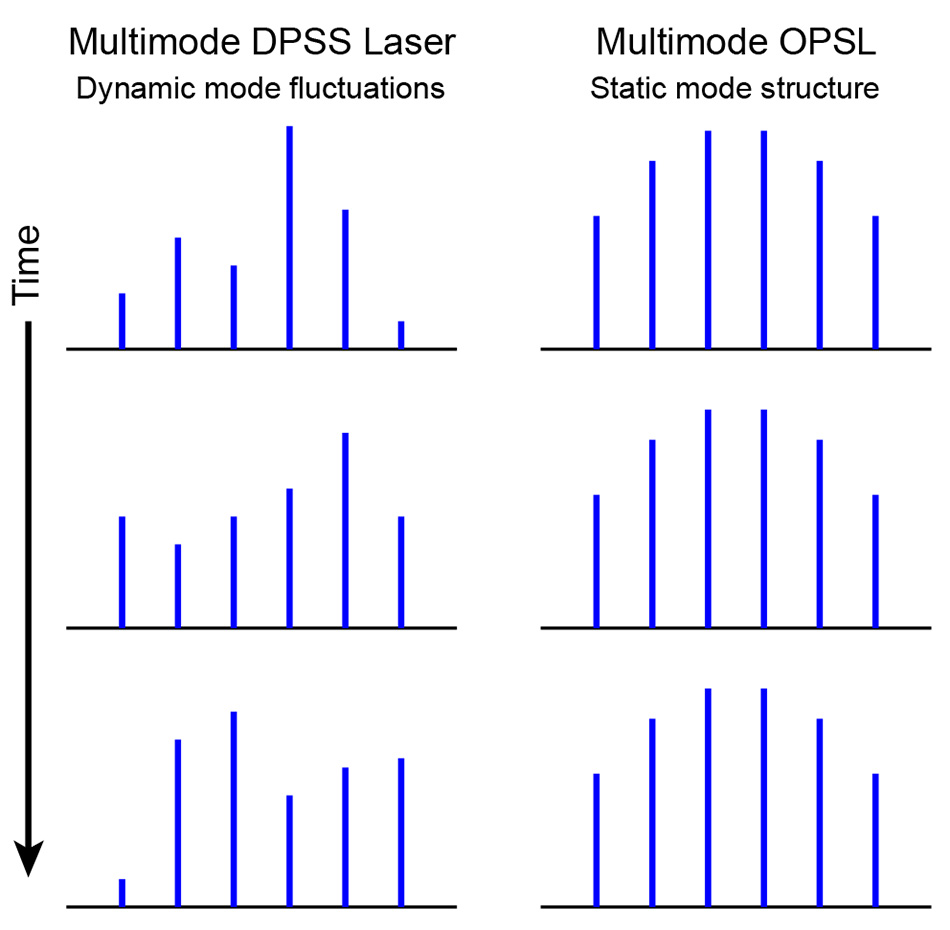
Figure 1: Longitudinal mode dynamics of DPSS lasers and OPSLs.
However, in the case of legacy technologies, such as ion and DPSS lasers, the division of the overall intracavity power between these individual modes is quite random and dynamic, with varying admixtures of these modes lasing over time and competing for the available stored gain as shown in figure 1. But because the intensity sum stays constant, multimode operation in ion lasers was quite suitable for most applications needing low amplitude noise.
In ion lasers and DPSS lasers, this dynamic competition between the different modes arises because the active laser medium has stored energy. In simple terms, the excited state of the gain medium has a much longer lifetime than the trip time for photons to circulate around the CW cavity. Specifically, the excited state lifetime in Nd-based DPSS lasers is microseconds, whereas the cavity trip time is only nanoseconds. Stored energy is actually an advantage for some pulsed laser applications because it enables a mechanism called Q-switching which generates very short and intense pulses. However, it limits how fast the laser can be modulated (turned on and off). And, just as important, it causes noise problems when using frequency conversion to generate harmonics of the fundamental, e.g., doubling the 1064 nm fundamental wavelength to generate CW green output at 532 nm.
Frequency Doubling Creates Green (and Ultraviolet) Noise
Both DPSS lasers and OPSLs generate their fundamental output in the near infrared, which is then frequency doubled to produce visible output, or frequency tripled to produce ultraviolet output using so-called non-linear crystals. These second harmonic generating (SHG) and third harmonic generating (THG) processes are highly dependent on intensity – the power per unit area in the SHG or THG crystal. With pulsed lasers, the peak power can be many orders higher than the average power, so efficient frequency doubling (and tripling) is easily performed downstream of the laser cavity, i.e., extracavity. But with CW lasers, the only way to obtain high intensity is to place the SHG and THG crystals inside the cavity, where the circulating power can be up to two orders of magnitude greater than the output power. And now the formerly innocuous mode noise becomes a real problem.
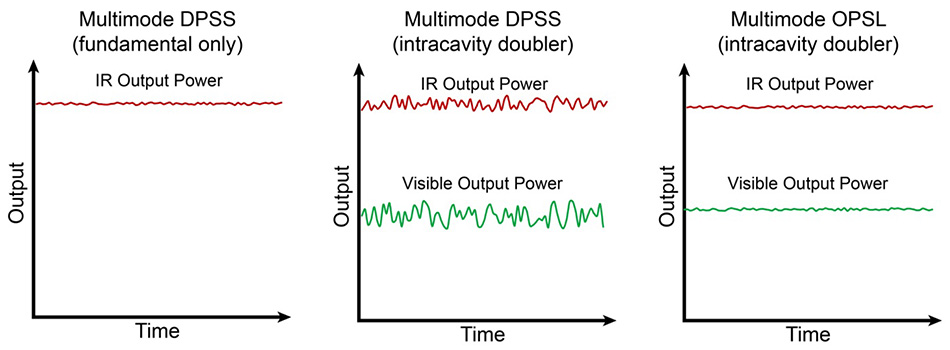
Figure 2: In a CW DPSS multimode laser, the total power is constant even though it is dynamically distributed between different longitudinal modes. When a doubling crystal is inserted in the DPSS cavity, it causes chaotic fluctuations in the total power. This noise mechanism cannot occur in OPSLs.
When a doubling crystal is inserted into the fundamental intra-cavity beam of a DPSS laser having multiple longitudinal modes, it creates chaotic intensity noise in both the fundamental and doubled output – see figure 2. The reason is that both second-harmonic generation (doubling the frequency of one longitudinal mode) and sum-frequency generation (adding the frequencies of two different longitudinal modes) are possible. Sum-frequency generation couples individual longitudinal modes and thereby enables direct dynamic interactions between longitudinal modes. The temporal dynamics from all the pair-wise interactions of longitudinal modes, whereby the intensity of one mode depends on the gain of another mode, generates significant intensity noise. This long-recognized phenomenon is called the “green problem,” [ref 1] since the first widely used CW lasers using intra-cavity doubling were green DPSS lasers, where the laser fundamental at 1064 nm is frequency-doubled to generate green output at 532 nm.
CW DPSS Lasers: Performance/Cost Trade-offs
Several methods have been used in CW DPSS lasers to address the mode noise problem. An early approach was to use an elongated cavity in order to split the power over a larger number of longitudinal modes. The idea is that the noise level is reduced by averaging the noise effect of many more modes. This “smearing” approach is sufficient for some applications, but for those that are particularly noise-sensitive, such as Carrier Envelope Phase (CEP) stabilization, it has proven inadequate. And of course, it can negatively impact applications that depend on monochromaticity, i.e., narrow spectral bandwidth.
A more rigorous approach is to actually remove the green noise at its source. The most direct way to do this in a DPSS laser is to make the laser operate on a single longitudinal mode using an optic such as an etalon. This requires active thermal stabilization of the cavity as well as the ability to lock the cavity length and etalon performance together using piezo mirror mounts and feedback electronics. This all adds cost and complexity.
Some commercial low-noise DPSS lasers are based on other active feedback noise reduction strategies. But in every case, there is an inevitable trade-off between noise, cost, and complexity.
OPSL – Delivering Low Noise Visible Output
With OPSLs, the gain dynamics are completely different. The gain medium is a semiconductor where pump light creates holes and electrons inside quantum wells. Radiative and non-radiative recombination of these charge carriers are both very fast processes. So in an OPSL, the effective upper state lifetime is a few nanoseconds or less, i.e. on the timescale of the cavity trip time. This has two benefits. First an OPSL can be directly modulated at speeds up to 100 kHz. And more importantly, the short upper state lifetime means there is no stored energy on the laser mode timescale – only instantaneous gain. Where the OPSL is operating on multiple longitudinal modes, the behavior of these cavity modes therefore is determined solely by the cavity, the gain just follows along. The distribution of power among these modes is thus stable with time.
Because the power distribution is completely stable, there is no noise due to non-linear coupling between the longitudinal modes when an intracavity doubling crystal is used to create visible output. The green problem simply does not exist in OPSLs because of the short upper state lifetime. Since there is no need for noise suppression mechanisms with their associated cost and complexity, there is no trade-off between performance and complexity (cost, potential failure modes). Of course, OPSLs can be designed for a single mode operation, and Coherent offers these for applications such as interferometry. But with OPSLs, single-mode is an option for these high coherence applications, not a prerequisite for low noise.
OPSL Delivering True-CW Ultraviolet Output
Frequency tripling can be used with DPSS and OPSLs in order to produce ultraviolet output. As with visible lasers, Q-switched DPSS lasers can use extracavity harmonic generation with excellent efficiency. This is the basis of several industrial nanosecond lasers produced by Coherent for precision materials processing applications. But with CW operation, the green noise problem manifests as a UV problem with increased severity because the tripling efficiency is determined by the third power of the focused intensity. For applications where quasi-CW output is acceptable, such as laser direct imaging of printed circuit boards, the DPSS laser can be mode-locked with a repetition rate of tens of MHz. An example is the Paladin series of lasers where the high peak power of the picosecond pulses means that extracavity tripling is very efficient. However for applications such as data storage and live cell sorting the pulsed output and/or high peak power of pseudo-CW operation can be a problem. Again OPSL technology provides an optimum solution without resorting to noise suppression mechanisms such as stabilized single-mode operation. For example, the Genesis 355 laser is now the accepted standard for the growing demand of flow cytometry applications requiring UV output, e.g., to excite endogenous fluorescence from DNA.
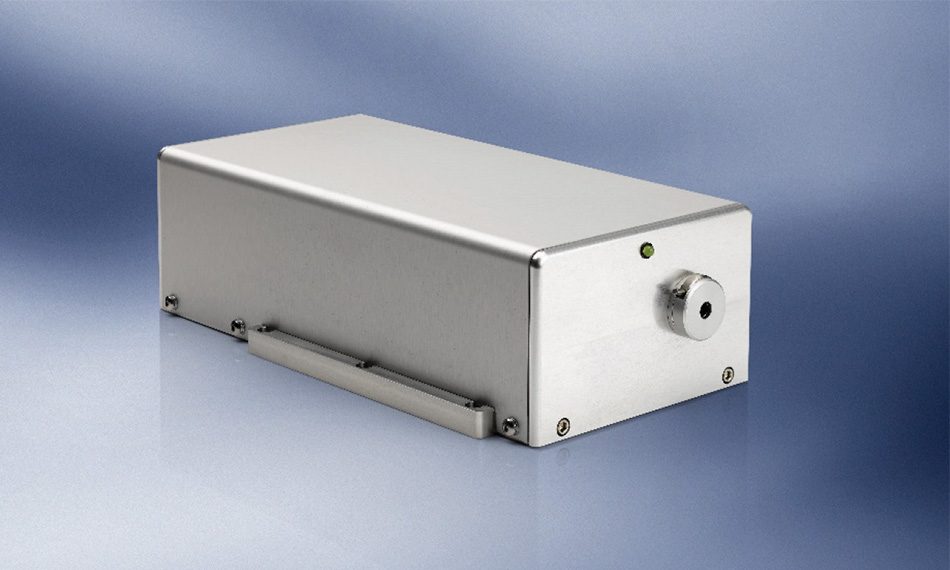
Figure 3: The Genesis 355 is a low-noise ultraviolet (355 nm) OPSL that delivers true CW output with very low noise for applications such as flow cytometry.
Summary
First generation, continuous wave solid-state lasers utilized DPSS technology. Obtaining visible and ultraviolet output with this technology is compromised by a phenomenon called green noise, leading to a trade-off between performance (low noise) and complexity (cost, and potential failure modes). The underlying mechanism responsible for this noise is completely eliminated in OPSLs. As a result, multimode visible and ultraviolet OPSLs provide superior noise characteristics without incurring any extra complexity or cost.
References
1. T. Baer, Large amplitude fluctuations due to longitudinal mode coupling in diode-pumped intracavity-doubled Nd:YAG lasers, J. Opt. Soc. Am. B, vol 3, 9, pp 1175-1180 (1986).