WHITE PAPER
New Material Enables kW-Class Faraday Isolators
KTF is a magneto-active crystal that offers substantially reduced thermal effects as compared to TGG. This enables optical isolators with better lifetime and performance in high-power laser systems.
Faraday isolators are one-way valves for light. They are often placed at the output of lasers and amplifiers to protect them from any light reflected back by downstream optics or surfaces. If this returned light re-enters the laser, it can cause output instability or even damage.
Faraday isolators rely on a magneto-active crystal; this is a material that, when placed in a magnetic field, will rotate the orientation of linearly polarized light. Terbium Gallium Garnet (TGG) has long been the standard magneto-active material for Faraday isolators operating in the visible and near IR spectrum. However, as the output power of industrial lasers continues to scale up, TGG’s inherent absorption and thermo-optical properties become increasingly disadvantageous. This can ultimately make the Faraday isolator the performance-limiting optical element in the laser system.
Now, Potassium Terbium Fluoride (KTF) has emerged as an alternative magneto-active material. It overcomes the limitations of TGG and can successfully operate at much higher laser powers. This document provides detailed information on the properties of KTF. It also reviews test results from a new series of Faraday Isolators intended specifically for high-power lasers – the Coherent Pavos Ultra series – that incorporate this material.
TGG And Its Limitations
TGG has long been the Faraday rotator crystal of choice for the 650 – 1100 nm spectral range for several reasons. For example, it can be grown with high purity. It has a high Verdet constant (a measure of the strength of its Faraday effect) and its cubic crystal structure and low intrinsic birefringence make it easy to achieve high polarization extinction without the need for sensitive alignment processes. And it is relatively low cost.
However, even the purest TGG encounters performance limits due to its bulk absorption. This absorption causes localized heating within the crystal which leads to three significant performance limiting factors.
The first of these is that the amount of polarization rotation changes as a function of laser power. This is because the Verdet constant of the crystal varies with temperature. And as the crystal warms, it also heats the surrounding magnets which changes their performance. The result is a degradation in isolation performance.
A second issue is thermal lensing. Since the crystal is typically held within a large permanent magnet, the crystal is difficult to cool directly. A Gaussian beam within the crystal produces a radial temperature gradient which causes a refractive index gradient. This has a lensing effect which is power dependent and will shift the focal position of the system. If the lensing is strong enough or asymmetrical, it can also reduce beam quality.
Another problem is thermally induced birefringence, again caused by a thermal gradient within the material. This affects the polarization of the transmitted light. This can reduce the performance of the isolator, as well as downstream optical components which rely on polarization.
Together, these three factors influence the power stability, beam quality, and focused spot position at the work surface. These all directly affect processing results and can therefore lower process consistency and reduce the size of the process window.
Sidebar
How Does a Faraday Isolator Work?
The operation of Faraday isolators is conceptually simple and is illustrated in this drawing. Linearly polarized light (coming in from the left) passes through a polarizer aligned with its polarization vector. It enters a magneto-active crystal which is within a magnetic field. This crystal rotates the polarization plane of the light by 45° (due to the Faraday Effect). The light passes through another polarizer aligned with the rotated polarization, and then out through the optical system to the process.
Any light returned from the system or process first passes through a polarizer which rejects any polarization which isn't oriented the same way the original isolator output. This filtered light then passes through the magneto-active crystal and undergoes another 45° rotation. This puts its polarization vector at right angles to the first polarizer which then rejects the remaining returned light.
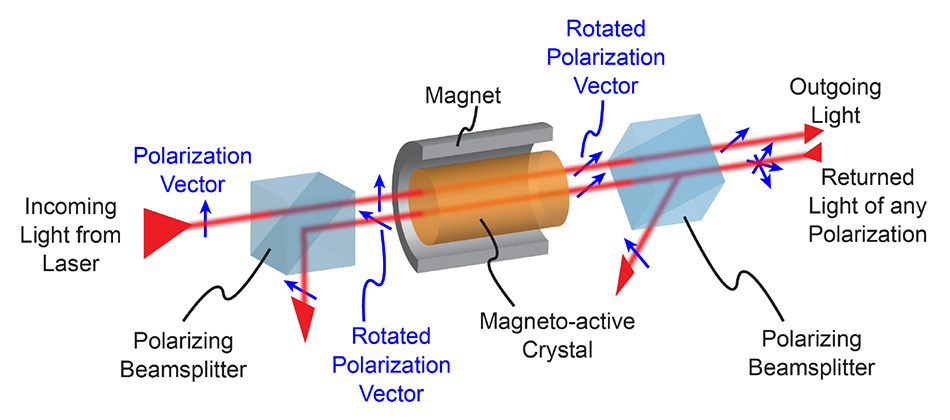
KTF And Its Benefits
KTF has a similar transmission range to TGG, as well as a comparable Verdet constant. Most importantly, as compared to TGG it possesses a lower bulk absorption coefficient (eight times lower), thermo-optic coefficient (15 times lower), and stress-optic coefficient. Together, these allow it to avoid the degradations in isolation performance, beam focus, and beam quality that plague TGG Faraday isolators when exposed to high laser power.
However, early KTF growth efforts produced boules with bubbles, inclusions, and issues of high scatter. These provided no net improvement in transmission over TGG.
Fortunately, continuous process refinements have now enabled higher yields of high-quality KTF at a reduced cost. As a result, KTF is poised to replace TGG in high-power Faraday rotators and isolators.
Pavos Ultra Series Experimental Data
Coherent Pavos Ultra Series Faraday isolators based on KTF have now undergone thousands of hours of lifetime testing with near-infrared, kW-class lasers. These tests clearly demonstrate that it delivers excellent isolation and beam quality, while maintaining performance over a long, continuous lifetime of use as required by industrial laser manufacturers.
The first graph compares optical isolation – the key performance metric of an isolator – as a function of laser power for TGG and KTF isolators. While TGG performs better at the lowest powers, its performance rapidly degrades as power increases. The stable performance of the Pavos Ultra isolator over the measured power range means that it can be relied on no matter how, and how long, the laser system is operated.
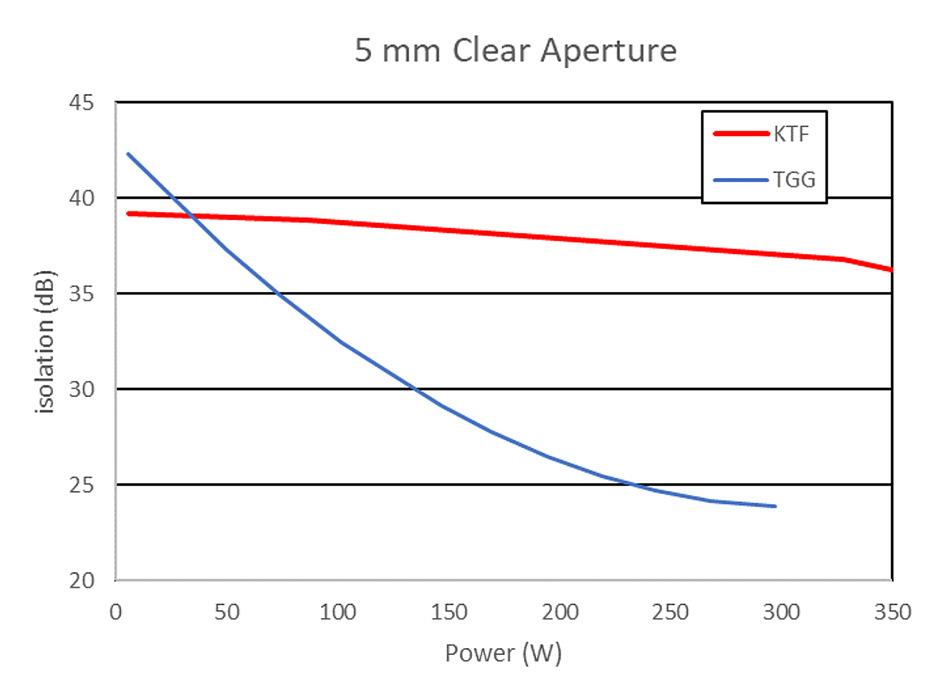
Figure 1: The isolation performance of KTF and TGG as a function of laser power.
The KTF isolator also maintains better beam quality than the TGG-based isolator. This is demonstrated in the beam profile measurements, shown for both types of isolators, at 6 W and 200 W of power.
Isolator Type |
6W |
200W |
TGG |
![]() |
![]() |
Pavos Ultra (KTF) |
![]() |
![]() |
Figure 2: Beam profile effects in KTF and TGG as a function of laser power.
A more quantitative measure of beam quality is provided using the M² metric. This is a ratio that compares the intensity profile of a measured beam to a theoretically perfect Gaussian beam. The next graph compares measured M² for both TGG and KTF isolators. Clearly, there is almost no degradation in beam quality for the Pavos Ultra isolator over the tested power range.
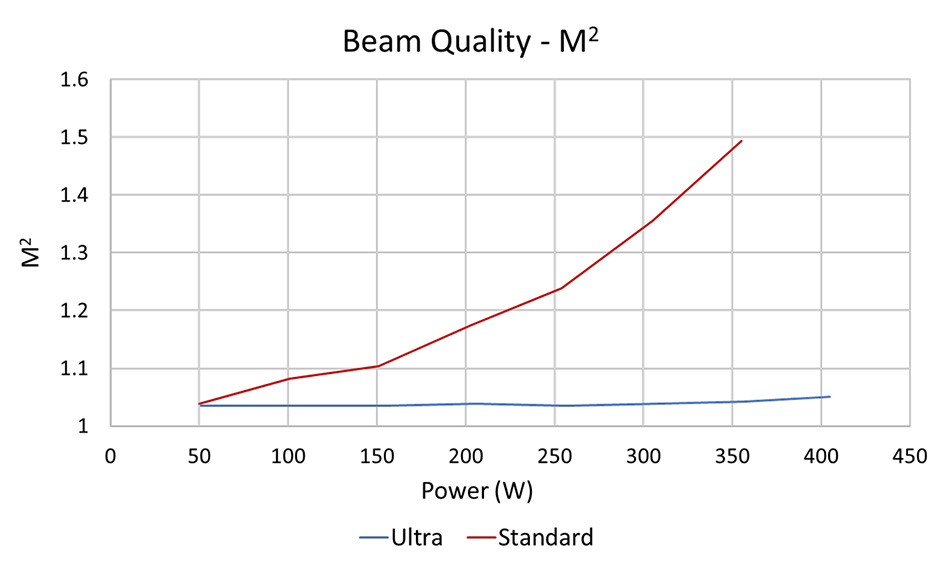
Figure 3: Beam quality in KTF and TGG as a function of laser power.
Focal shifting is one of the most significant problems with using Faraday isolators at high power. This is because even if the laser system continues to operate without damage or even instability, a focal shift can degrade process results.
Even though the thermal conductivity of TGG is an order of magnitude higher than for KTF, experimental results clearly demonstrate significantly lower thermally related focal shifts and better beam quality when compared to TGG at equivalent power levels. Test results are provided in the next graph.
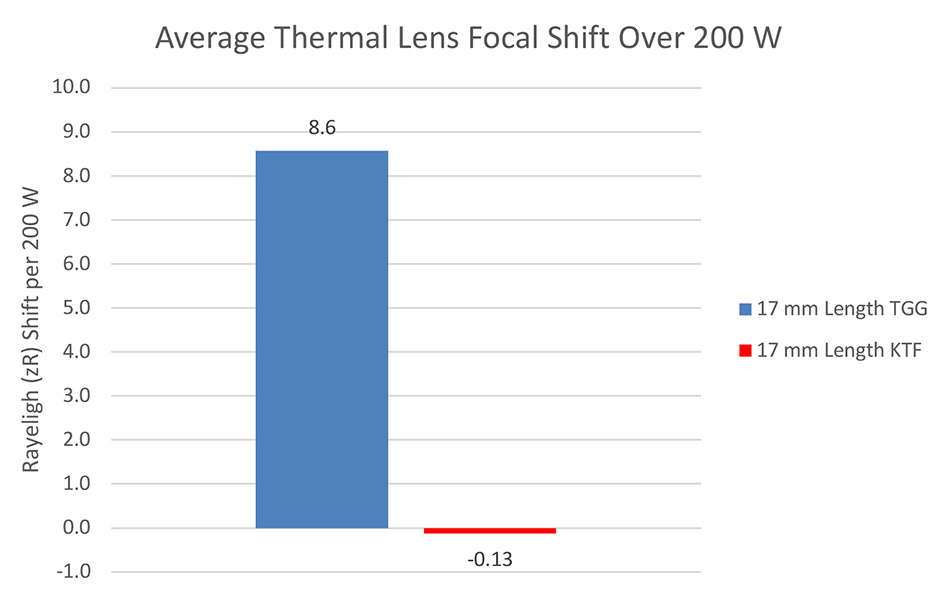
Figure 4: Focal shift over a 200 W laser power change for KTF and TGG isolators.
What isn’t seen in the graph is that the small focal shift experienced with KTF is also linear. That means it’s likely that the measured shift can be extrapolated to provide the expected focal shift at higher power levels.
Another important point to note from the last plot is that KTF demonstrates a negative focal shift. Specifically, beam divergence increases with temperature, as opposed to the self-focusing which occurs in absorbing optics with a positive shift.
This can actually be beneficial when KTF is used together with other positive shifting optics (such as fused silica components). Specifically, the negative shift of the KTF will partially compensate for the positive shift of the other components, resulting in a lower net focal shift for the entire system.
For instance, the Coherent 4 mm aperture PAVOS Ultra isolator uses two fused silica polarizing beamsplitter cubes and a KTF crystal. Each beamsplitter has approximately a 0.3 zR/kW focal shift. The KTF crystal has an average focal shift of -0.6 zR/kW. The result is generally a negligible focal shift for the complete isolator.
The long-term performance of Coherent PAVOS Ultra series isolators has also been examined. Specifically, these isolators were tested within prototype laser cavities at Coherent over usage intervals of 1800 – 3000 hours.
The incident power at the KTF crystal was 2.7 kW with an approximate beam diameter of 800 µm. This translates into a power density of just over 130 kW/cm². The graph shows that the cavity remained stable over the entire test period of 1800 hours. All of the jumps or changes were due to adjustment of other system components outside of the KTF rotator. Maintaining this stability required that the beam quality stay constant.
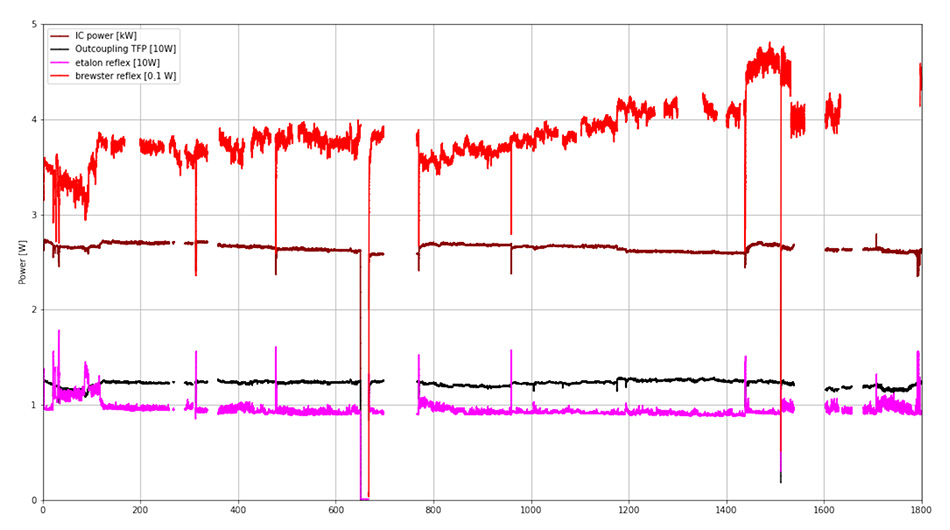
Figure 5. Long-term operating stability of the KTF-based Coherent Pavos Ultra isolator when exposed to high laser power.
Conclusion
While TGG remains the first choice magneto-active crystal for lower-power Faraday isolators and rotators, its inherent absorption and thermo-optical properties limit its use with higher-power lasers. By adopting KTF as the new standard for high-power Faraday isolators, laser manufacturers will be able to remove the constraints imposed by TGG and focus their efforts on improving performance in the rest of the system.