ホワイトペーパー
THz-Ramanアナライザーの新たな応用例
概要 - ナノ構造解析
THz-Raman™分光法は、比較的新しい分析手法であり、特別な試料調製を必要とせず、試料のナノスケール構造に関する明確かつ定量的なデータをリアルタイムで提供する、光学分光法の中でもユニークな方法です。 したがって、機能的性能が試料の化学的性質だけでなく、そのナノスケール構造にも依存するような、ほぼすべての研究・開発、加工方法モニタリング、QC/QA用途に理想的なツールです。 具体的な用途には、粒子径が重要な意味を持つコロイド、結晶性や層厚が物理特性(引張強度、流動性など)を決定するポリマー、分子配列が光透過率を決定する液晶ディスプレイ技術、ドットサイズが性能を決定するディスプレイや太陽電池(ソーラー)などの光応用、さらに膜厚が光機能を決定するペロブスカイト膜などの2次元材料への応用などが挙げられます。 特別な試料調製や複雑な装置組込みを必要とせず、シンプルなプッシュボタン式の分析装置を用いて、ガラス窓越しにでも測定できることは、粉末X線回折(PRXD)などの非分光技術に対するTHz-Ramanの大きな優位点です。 以前のホワイトペーパー、THz-Raman定量分析の医薬品への応用で詳細に述べたように、例えば多形や共結晶が投与レベルに直接影響を与えるなど、THz-Ramanが医薬業界で急速に受け入れられてきています。 このホワイトペーパーでは、他の産業や技術における興味深い新しい用途をいくつかご紹介します。
- ポリマー*とコポリマー*
- 半導体ナノ結晶(量子ドット)
- ペロブスカイト薄膜*
- 2D素材*
- 発光性有機結晶(ルブレン)
- 液晶
- コロイド
- 粘着剤の硬化*
- 不正原料の出所表示
- 酸素リークモニタリング
*本ホワイトペーパーに掲載されているデータ例
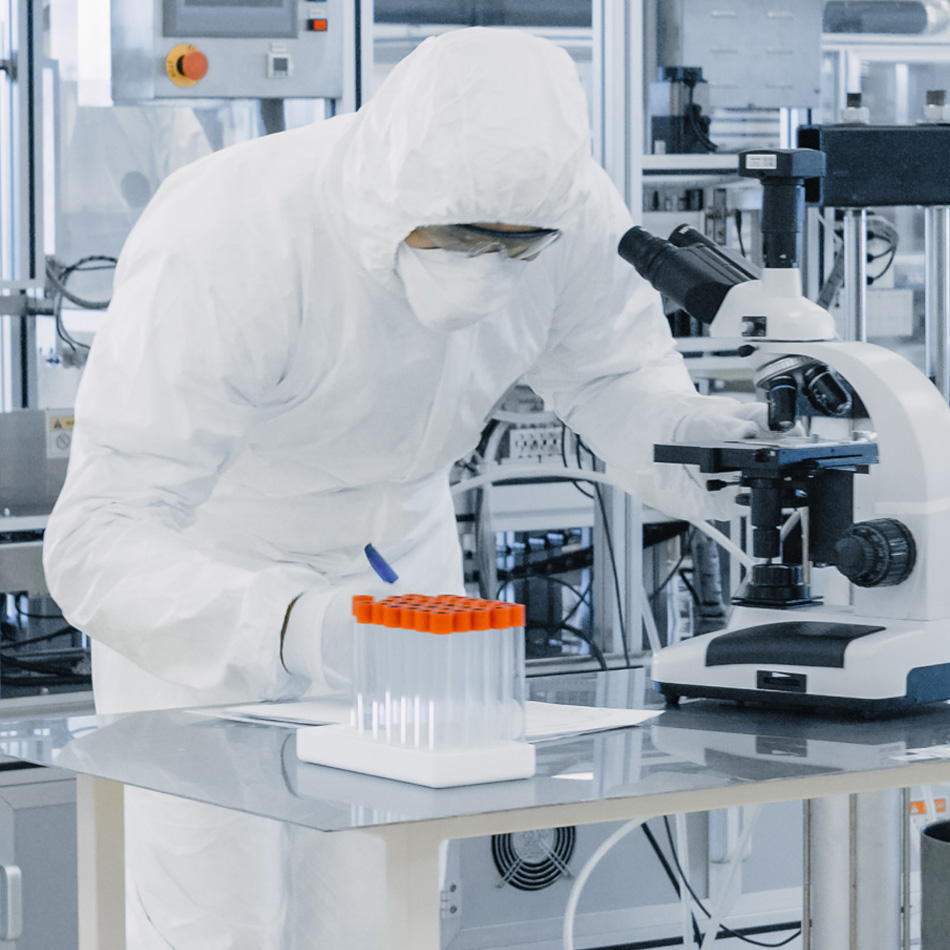
THz-Ramanの背景について
THz-Raman分光法(低周波Ramanとも呼ばれる)は、振動分光法の一種です。 FTIRや従来のラマンが分子内の振動を検出するのに対し、THz-Ramanは0.15 THzから6 THz(すなわち、5 cm-1から200 cm-1)という非常に低い周波数領域で自然に発生する大規模振動を検出します。これらの振動には、固体の格子振動、すなわちフォノン、タンパク質やウイルスなどの生体分子の変形、ポリマー鎖の運動、コロイドなどの大きな粒子の振動、薄い層の「呼吸」運動などがあります。 つまり、これらの振動モードは、試料の大きさ、形状、コンフォメーション、秩序、局所相(結晶と非晶質)を含む情報を提供します。 さらに、アンチストークスとストークスシフトのラマンピークの比は、これらの低次元振動状態のポピュレーションを示し、試料のボルツマン温度データを得ることができます。
THz-Raman分光法については、以前のホワイトペーパー、THz-Raman分光法の紹介で包括的に解説しています。 THz振動の研究は、古くからユニークな情報を豊富に提供することが知られていましたが、THz-Ramanは、弱いRaman散乱光と元の波長の強い(レイリー)散乱光とを分離する問題があり、歴史的に困難なものでした。 Ondax(現Coherent)の技術者は、ボリュームブラッググレーティング(VBG)と呼ばれる新しいタイプのガラスフィルターでこの課題を解決しました。 レーザに関する専門知識と垂直統合により、Coherentは包括的なTHz-Ramanツールを提供できるようになりました。 その範囲は、既存のRaman分光器をTHz領域に拡張するコンポーネントからターンキーモジュール、顕微鏡モジュール、従来のサンプルバイアル用のベンチトップアナライザー、粉末や液体のアットライン分析用の液浸プローブ、研究グレードの分光器一式までと多岐にわたります。 この製品群は、研究室、R&Dアプリケーション開発、プロセスモニタリング、サンプルQC/QAのための共通で使いやすいプラットフォームとなります。
以下に挙げる様々な例は、THz-Ramanで得られるユニークなデータから恩恵を受けることができる数多くの新しい用途のほんの一例です。
ポリマー
高密度ポリエチレン(HDPE)の魅力は、そのコスト競争力だけでなく、幅広い汎用性にあります。 例えば、HDPEの構造特性は、レーザアニーリングによって、ラメラの厚みと呼ばれるある臨界距離の長距離秩序に影響を与えることができます。 レーザアニーリングの方法を変えると、ラメラの厚みや結晶化度が変化し、プラスチックの機械的性能に直接影響を与えることになります。 この可変性により、ミルクジャグやボトルキャップからプラスチックバッグやプラスチック製材料に至るまで、様々な用途に使用することができます。
従来の化学物質の同定を目的とした分光法では、これらの異なる種類のHDPEはすべて同じ材料であるため、分析には特に役立ちません。 しかし、THz-Ramanでは、結晶化度やラメラの厚みといった相の詳細の影響を強く受ける低周波モードのデータが得られるため、この種の分析に最適です。 また、THz-Ramanは試料調製が不要で、非接触のツールであるため、オンラインまたはアットラインでリアルタイムにポリマー構造の変化をモニタリングするための理想的なソリューションとなり得ます。
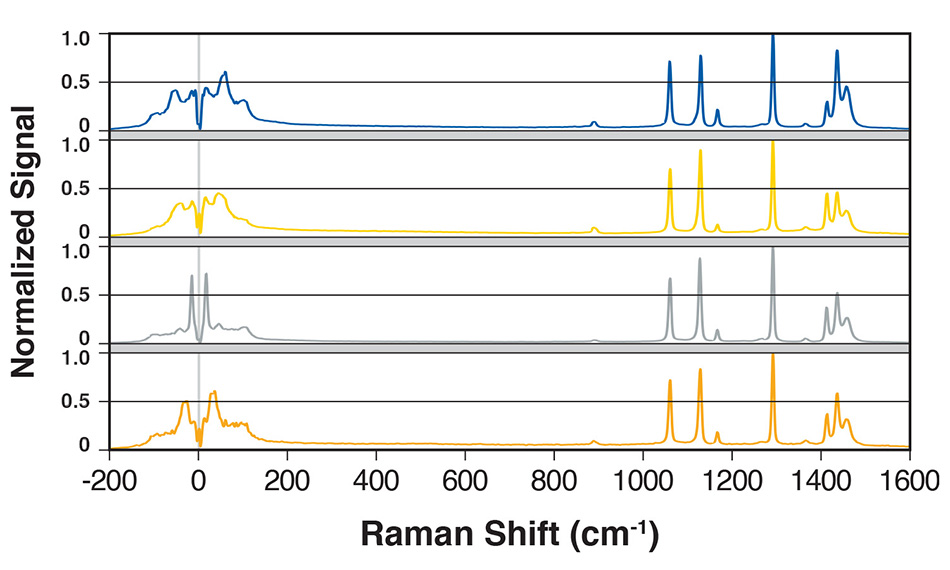
図1: ラメラの厚さが異なる4種類のHDPEサンプルの完全なRamanスペクトル。 大きなスペクトルの違いは、THz(<200 cm-1)領域のみに見られます。 出典[1]。
最近、Coherentの分光アプリケーションラボで行われたHDPEサンプルの研究では、CoherentのTR-Micro-785アナライザーを用いて、この用途に対するTHz-Ramanの有効性が確認されています[1]。 図1は、ラメラの厚さが異なる4つのHPDEサンプルの本研究で得られた典型的な完全なTHz-Raman分光スペクトルです。 予想通り、これら4つの試料のRaman「化学指紋」スペクトル(2000 cm-1)は驚くほどよく似ていることがわかります。 しかし、縦波音響モードを包含するTHz領域(<200 cm-1)では、ピーク位置とバンド形状に明確な違いが見られます。
コポリマー
コポリマーは2種類以上のモノマーから作られるポリマーで、バイオポリマーは最も一般的に市販されているタイプです。 自動車のタイヤからストレッチ素材、一般的なプラスチック部品まで幅広く使用されています。 ポリ[(R)-3-ヒドロキシブチレート-co(R)-3-ヒドロキシヘキサノエート](PHBHx)は、バイオベースの完全に生分解性の半結晶性熱可塑性コポリマーで、商業用途向けに他の様々な魅力的な特性を備えています。 最近発表されたNodaらによるApplied Spectroscopy [2]の研究では、中周波RamanとTHz-Raman測定を組み合わせて、PHBHxの等温結晶化について研究しています。 中周波のCO伸縮モードとTHz格子モードの測定を組み合わせることで、ヘテロモード相関解析を行い、2次元分光データを提供することができました。 (ちなみに、Coherent製プローブヘッドの高い光スループットとフィルタリング効率(レイリーブロック)により、中波長Raman分光器に通常組み込まれているホログラフィックノッチフィルターを完全に省略することができたと本書で述べています)。
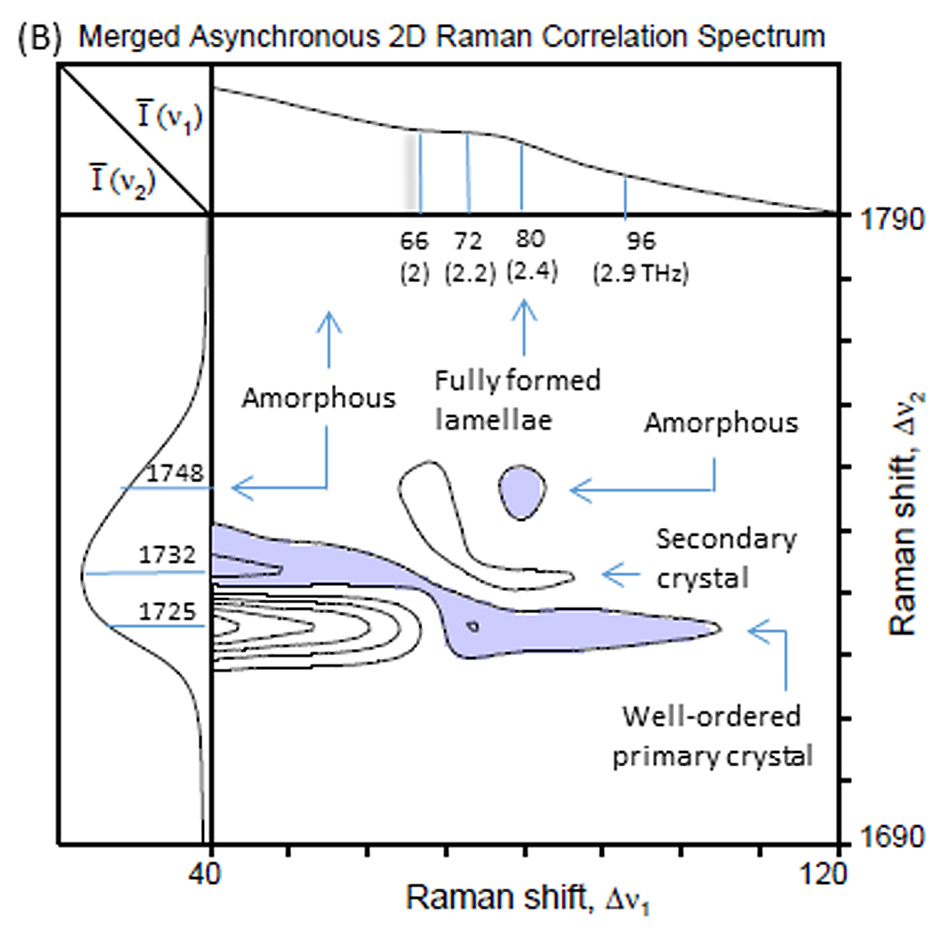
図 2: PHBHxの結晶化に関与する異なる相の特徴を示す非同期2次元ラマン相関スペクトル。 許可を得て[2]より転載。
図2は、本研究で得られた非同期2次元Raman相関スペクトルをマージした例です。 66~96 cm-1ウィンドウの低周波のヘリックス間およびヘリックス内の振動モードに関する既知の分光学的情報と合わせて、スペクトルの特徴をPHBHxの異なる状態 (非晶質、整然とした初晶、二次結晶、完全形成ラメラ)に明確に割り当てることができるようになりました。 これらのスペクトル特徴の変化をリアルタイムで追うことで、結晶化過程の詳細なシーケンスを初めて追跡することができました。 このようなデータを活用することで、共重合体の調製や結晶化を監視・操作し、弾性率などの物性を決定論的に最適化することができます。
金属ハロゲン化ペロブスカイト(MHP)多形体
ペロブスカイト薄膜は、太陽電池、LED、光検出器などの光応用に大きな可能性を持っています。 例えば、ハイブリッド型ハロゲン化鉛ペロブスカイトは、すでに20%以上の太陽光変換効率を実証しています。 しかし、その光特性をより詳細に理解し、効率、信頼性、寿命の面でフィルムやデバイスを最適化するためには、 まだ多くの研究が必要です。 このため、最近Journal of Materials Chemistry C誌に掲載されたYangらの研究[3]では、Coherent THz-Ramanベンチトップモジュールを用いて、ハイブリッドハロゲン化鉛ペロブスカイト、特にCsPbIXBr3-XとMAYCs1-YPbI3の低周波振動モードが研究されています。 このタイプモードは、電子-フォノン結合を通じて材料の光物性に影響を与えるため、この種の情報は重要です。 Nature Communications誌に掲載されたGuoらによるハロゲン化鉛ペロブスカイトの別の研究[4]では、低周波Ramanが、キャリア再結合発光に対する強い格子非調和性の結果の決定に役立っています。
図 3: 室温で収集した低周波Raman分光スペクトル。 許可を得て[3]より転載。
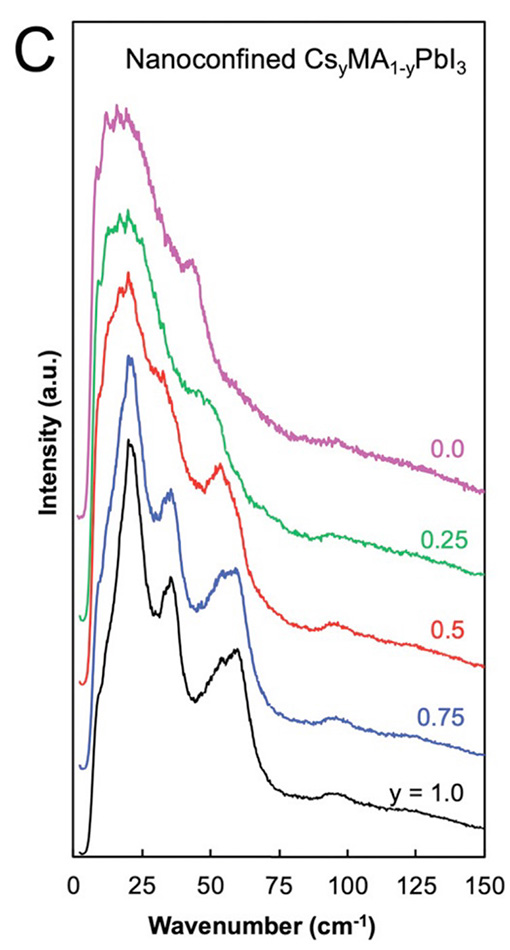
これらの物質のうち、γ-CsPbI3などの興味深い多形は、室温で準安定であり、湿気によって劣化し、バンドギャップ以上のエネルギーのレーザ光にさらされると劣化するものがあります。 (幸いなことに、THz-Ramanの信号強度は高いため、より低いレーザ出力で使用することができます。) そこで研究者らは、安定化テンプレートを用いて、陽極酸化アルミニウム(AAO)テンプレートの直径20~250 nmの円筒形ナノ穴に、溶液中の様々なペロブスカイト材料を蒸着させました。 この外部安定化により、THz-Ramanモジュールを用いた研究の延長が可能になりました。 このモジュールには、試料からの蛍光を避けるために976 nmのレーザ光源が搭載されていますが、標準的なシリコンベースのCCD検出器が使用されています(図3参照)。
この研究では、相転移をリアルタイムで観察するなど、多くの興味深い観察と結論が得られています。 特に、化学的置換により、これら一連の金属ハロゲン化ペロブスカイトの格子振動のエネルギーを支配する主要因は、イオンレーザの性質ではなく、格子サイズであることが示されました。 具体的には、格子間隔の増加とともにピーク位置が低波数側にシフトすることがわかりました。 このことから、フォトニックデバイスの効率に影響を与える電子-フォノン結合の相互作用を操作するためには、格子間隔の考慮が重要な要素になることがわかります。
接着剤の硬化をリアルタイムにモニタリング
製造工程でエポキシ樹脂を確実に硬化させることは、ほとんどの製造業において重要な検討事項となっています。Raman分光法は、エポキシ樹脂の架橋プロセスを監視するために採用されている方法の1つです。 これらのRaman測定のほとんどは、500 cm-1から2000 cm-1までの化学指紋領域に限られており、実際にはスペクトルのピーク変動は非常に小さいものです。
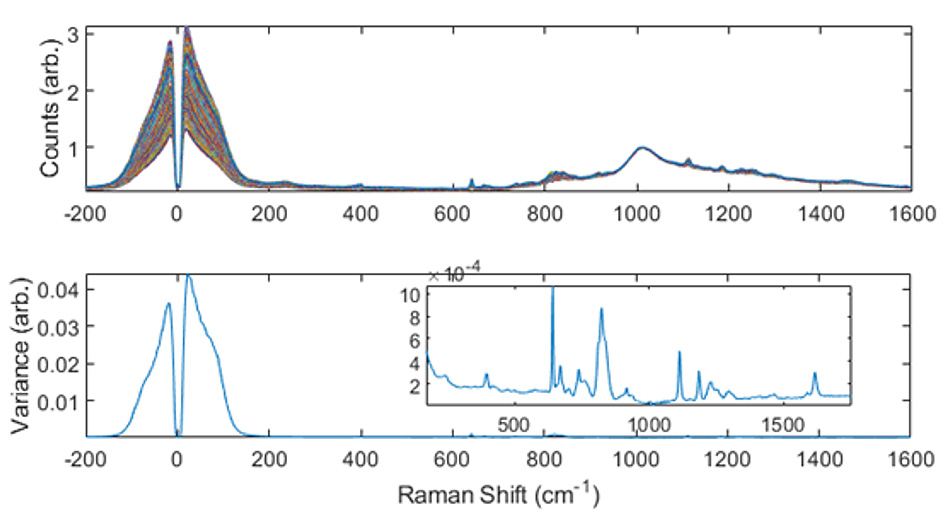
図 4: (上)「5分」エポキシ樹脂の25分間に記録された多数のRamanスペクトルのフォールスカラーコンポジット。 (下)このデータから得られたスペクトル分散のプロット。
最近、Coherentのアプリケーションラボで、硬化過程をモニターするためのTHz-Ramanの有用性を評価する研究が行われました。 市販のエポキシ樹脂を数種類、硬化時間を5分から120分まで指定して検討しました。 手動で混合した後、Coherent TR-Probe(808 nmレーザ搭載)とステアラブル非接触光学アクセサリーを倒立顕微鏡として使用し、各サンプルのスペクトルを取得しました。 各エポキシタイプについて、混合などのランダムな変動を考慮し、複数のサンプルを実行しました。
図4は、この研究で得られた代表的なデータです。メーカーによると、公称5分のセットタイムと1時間の硬化時間を持つエポキシの場合です。 上図は、25分間に1分間隔で取得したスペクトルをフォールスカラーで表示したものです。 低周波(200 cm-1未満)において、その差が非常に大きいことは、一目見ただけで明らかです。 これらのRamanシグナルは、架橋中のエポキシのバルクせん断モードとブリージングモードの変化と相関していると思われます。 各スペクトル系列を正規化し、スペクトル分散のプロットに変換すると、その差はさらに顕著になります。 その典型的な例を図4(下)に示します。 化学指紋領域(すなわち「従来の」Ramanスペクトル)の強度変化(分散)は、低周波の分散と同様の大きさにするために、2桁のスケールアップが必要であることに注目してください。
数種類のエポキシ樹脂を詳細に分析した結果、20 cm-1と85 cm-1におけるラマン強度の比が、エポキシ硬化プロセスのキネティクスを監視するための有望な指標となることが示されました。 図5は、異なるエポキシ樹脂のサンプルについて、この比率をプロットしたものです。このサンプルは、メーカーによれば、公称120分のセットタイムと24時間の硬化時間を持つ「海洋」タイプの接着剤です。 この研究では、低速硬化と高速硬化の異なるエポキシ樹脂についても同様のデータが得られ、好気性と嫌気性の両方の硬化条件におけるエポキシ樹脂のモニタリングに、低周波Ramanデータが幅広く利用できることがさらに確認されました。非破壊、非接触、高速というTHz-Raman測定の特性により、これらの接着剤やその他の工業用接着剤の完全硬化時間や硬化時間のカスタマイズプロセスを開発し、高品質の接着を確保しながら時間を短縮することが容易になります。
図5: 硬化時間120分の手動混合エポキシ樹脂の20 cm-1および85 cm-1におけるラマン強度の比率のリアルタイムプロット。
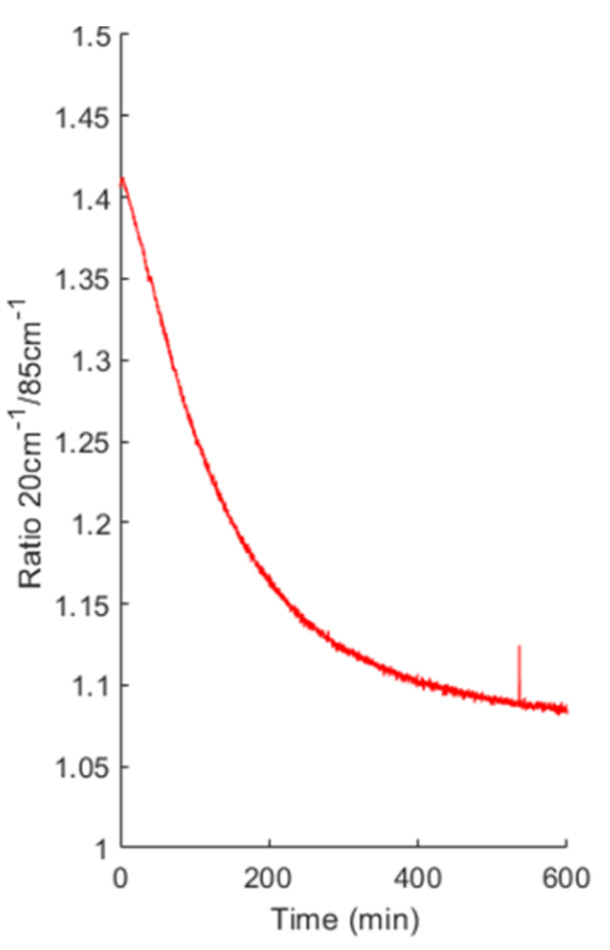
2次元材料における層特性
単層あるいは数層の材料は、しばしば2次元材料と呼ばれ、バルク相とは劇的に異なる性質を持っています。 このうち、遷移金属ダイカルコゲナイド(TMD)を用いた2次元材料群は、ユニークで有用な電子・光特性を示します。 しかも、これらは垂直方向に積層された層間の相互作用に大きく支配される調整可能な特性であり、層の数や配向に影響されます。
ファンデルワールスヘテロ構造と呼ばれるタイプの層状2次元系への関心は、2018年に科学者が2つの層の間に1.1°のいわゆる「マジックアングル」のねじれを持つデュアルグラフェン層のサンプルを作製できたことによりさらに高まりました。 理論的に予想されたとおり、わずかな電圧でオン/オフできる超伝導の領域が見つかったのです。
応用科学者やデバイスエンジニアが、このような興味深い2次元材料の例を数多く研究していく上で、層間の相互作用を分析し監視するためのシンプルなツールは非常に貴重な存在となります。 THz-Ramanによる解析は、まさにそのようなツールであることを証明しつつあります。 従来のRaman分析では、層の化学的な詳細を知ることができますが、RamanをTHz領域に拡張することで、層間振動を分析するための直接的な測定ツールを得ることができます。 この振動の特性から、層間の相互作用力を定量的に把握することができます。 THz-Ramanは、このデータを得るためのシンプルで非破壊的な手段を提供する唯一の分析モダリティであり、サンプルの前処理を必要としないため、直接その場で測定することができます。
2次元材料におけるこの低周波の層間振動には、2つのクラスがあります。 層間距離をほとんど変えずに層間が相対的にスライドする振動を、せん断振動またはせん断モードと呼びます。 これに対し、層間に垂直な動きを伴う振動、すなわち層間距離を変化させる振動は、ブリージングモードまたはブリージング振動と呼ばれます。
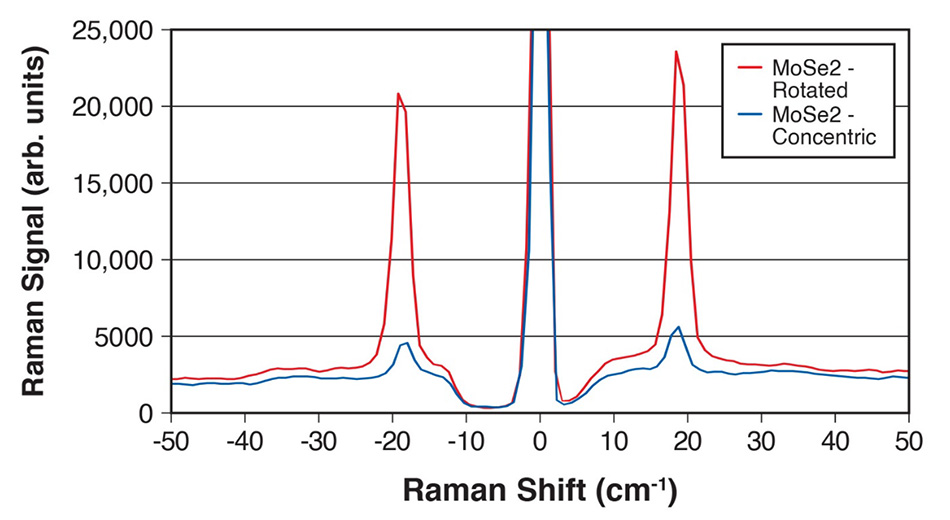
図6: 2層構造MoSe2材料の正規化THz-Raman™スペクトル。18cm-1のせん断モードピークに対応するピークのシフトと大きさの変化を、回転した層配向(赤)と同心円(青)で示したものです。 許可を得て[4]より転載。
層数が2層から増えるにつれて、層間振動の周波数は増加し、プラトー値になります。 その結果、低周波の振動データは、層の積層構成だけでなく、層数を特徴づける指紋の役割を果たします。 最近発表されたPuretzkyらの研究(ACS Nano誌[5])は、この方法でTHz-Ramanを用いて、2層および3層のMoSe2およびWSe2結晶の形の2次元金属ダイカルコゲニドを調査したものです。 本研究におけるRamanスペクトルは、Coherent TR-MICRO-532 THz-Ramanシステムに1段分光器(分光分解能1.5 cm-1)を接続して532 nmで励起し、収集されました。 図6に、MoSe2二層膜の2つの配向(回転と同心円)に対するせん断モードの規格化THz-Ramanスペクトルの例を示します。
ピークが18 cm-1(すなわち0.54 THz)に集中していることに注目してください。これは非常に低い周波数であり、他の手段では検出が極めて困難なものです。
その他の用途
このほかにも、試料のナノスケール構造や局所的な位相が機能に直接影響を与える用途は数多く登場しています。 LEDなどのフォトニックデバイスに用いられる半導体量子ドット、一部のディスプレイに用いられる発光性有機結晶(ルブレン、テトラセンなど)および液晶、コロイド懸濁液など、その種類は多岐にわたります。 また、機能的なインパクトよりもトレース解析が主目的の用途もあります。 そのひとつが、THz-Ramanが酸素を高感度で検出できることを利用した、石油およびガスパイプラインの空気漏れの検出です。 もう一つは、合成カンナビノイドなどの麻薬の痕跡を見つけて、出所を特定することです。 市販の材料の偽造品検出や防止も、THz-Ramanで改善することができます。 このような応用が可能になるのは、同じ化学物質がTHz-Ramanスペクトルを持つことで、合成経路や成分、処方を特定し区別したり、環境や保存状態(熱、湿度など)に関連した変化を明らかにしたりすることができるからです。 これらの要因はそれぞれ、分子構造に「サイン」や「指紋」を残し、それが水紋のように作用して、法医学専門家が発生源の絞り込みや探索を加速させるのに役立つことがあります。
概要
THz-Ramanは、これまで面倒なX線回折や他の複雑な方法を必要とした構造および位相データを提供する、新しく利用可能な分析モダリティです。 しかし、非接触、高速分析、特別な試料調製が不要など、光学分光法の使いやすさが備わっています。 バルク、微量、2次元の材料分析用途において、材料のナノスケール構造、相、秩序・無秩序に機能が依存するあらゆる用途に独自の適応性があります。
参考資料
1. Coherent, Inc., Structural Changes in Polymers, Application Highlights, 2017
2. I. Noda et al, Two-Dimensional Raman Correlation Spectroscopy Study of Poly[(R)-3-hydroxybutyrate-co-(R)-3-hydroxyhexanoate] Copolymers, Applied Spectroscopy. Vol 71, Number 7, 2017
3. Y. Yang, Probing lattice vibrations of stabilized CsPbI3 polymorphs via low-frequency Raman spectroscopy, J. Mater. Chem. C. Vol 8, 8896, (2020)
4. Y. Guo et al, Dynamic emission Stokes shift and liquid-like dielectric solvation of band edge carriers in lead-halide perovskites, Nat Commun 10, 1175 (2019).
5. A.A. Puretzky et al, Low-Frequency Raman Fingerprints of Two-Dimensional Metal Dichalcogenide Layer Stacking Configurations, ACS Nano, Vol 9, Number 6, 6333 (2015)