白皮书
双光子显微成像激光调制解决方案
综述<
自从双光子激光扫描荧光显微镜方面的开创性著作(登克等人,1990 年)于 1990 年发表以来,该技术已经从激光技术的巨变中受益。 这些变革进一步推动了这种最初从物理实验室中开发的技术向细胞生物学、疾病研究和高级神经科学成像领域的渗透。
一体式可调谐钛宝石激光器在 2001 年左右开始了这一趋势。几年后,激光器中增加了自动色散控制功能,以优化显微镜样品平面的脉冲持续时间。 随着在超过钛宝石激光器上限的波长下可激发的探测脉冲变得更加成熟和高效,2010 年之后,激光器公司转向了光学参量振荡器来满足对多颜色维度、更深成像和更少光损伤的需求。
在本文中,我们将讨论这一演变的下一阶段,即快速功率调制在激光系统中的集成,并讨论这个阶段将如何实现更快的设置时间、最高的性能和更低的拥有成本。
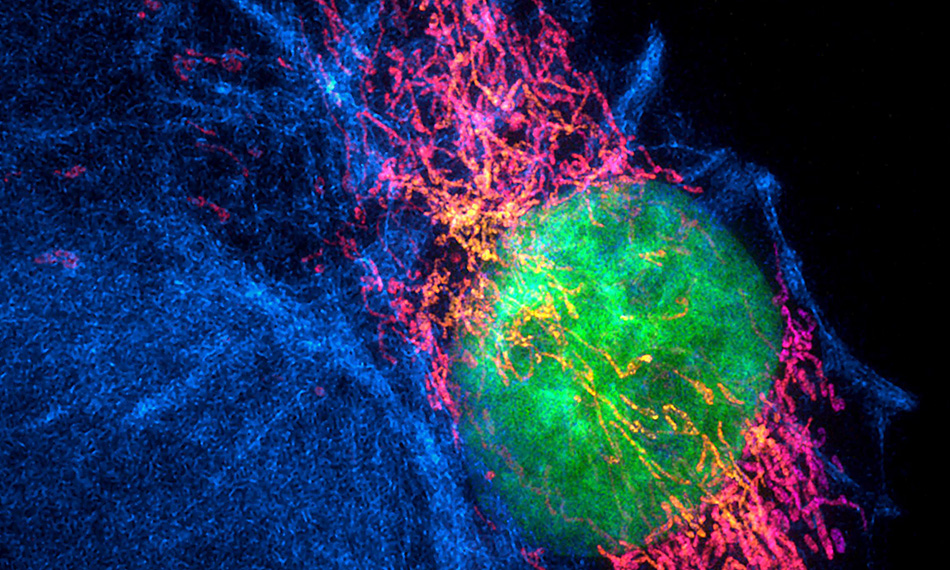
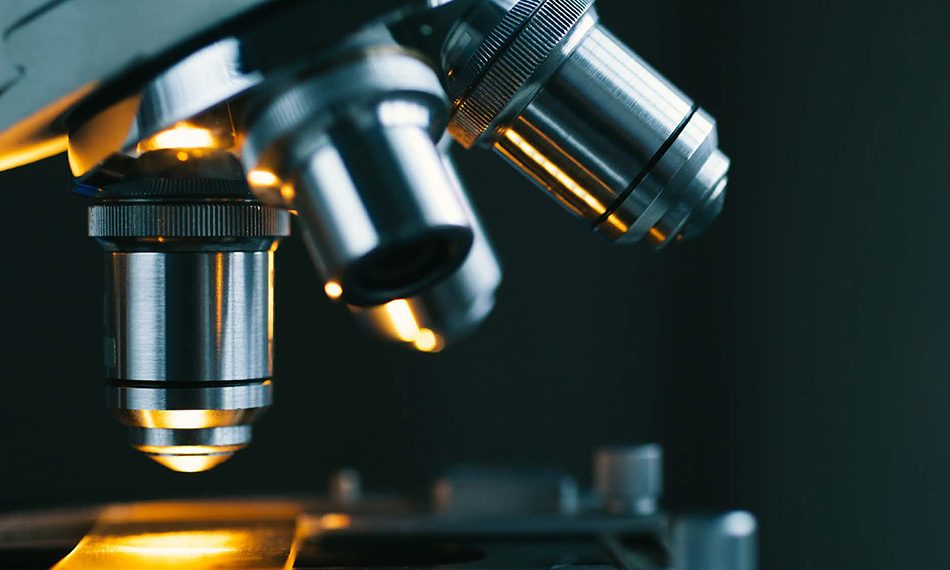
“在激光系统中集成快速功率调制可缩短设置时间、提高性能并降低拥有成本。”
双光子显微成像中的激光功率控制要求
在最简单的显微镜中,可以通过添加相位延迟波片和偏振分析仪来实现对激光功率的连续控制。 通过旋转波片,激光功率透过分析仪的透射率通常可以从 0.2% 透射率变为 99% 左右。 例如,通过电动旋转波片,该过程可以自动改变显微镜中成像平面的功率,以均衡不同深度帧处的聚焦积分通量。
不过,大多数现代激光扫描双光子显微镜都需要更快的调制速度。 例如,对于仅应在单个方向上进行数据采集的光栅激光扫描应用,在“反激操作”期间必须消隐激光以避免不必要的荧光激发或光漂白。 如果是共振振镜扫描仪,得到的上升/下降时间可能短至几微秒。 在这一领域,必须考虑光调制方法。
电光调制
电光调制器 (EOM) 利用普克尔斯效应,通过对光束施加相位延迟来调制激光功率。 在电光调制器中,通过施加电场,在非中心对称的晶体中诱发双折射。 和以前一样,使用偏振分析仪来完成调制器设置。
可以在纵向激发几何结构中配置普克尔斯盒,以适应具有相对较短晶体的较大光束。 在这种情况下,典型的 ½ 波电压(即偏振旋转 90 度所需的电压)约为 6 kV,这在 2P 显微镜的速度和占空比下难以实现。 因此,大多数成像配置采用横向电场几何结构,使用更长的晶体,这会显著降低半波电压。 晶体通常采用 2 种或更多种串行配置进行部署,彼此相对旋转,以进一步降低所需的开关电压并补偿热负载效应。
必须注意通过晶体校准和偏移(偏置)电压调整来优化脉冲对比度(最小与最大发射功率的比率)以获得优异的图像对比度。
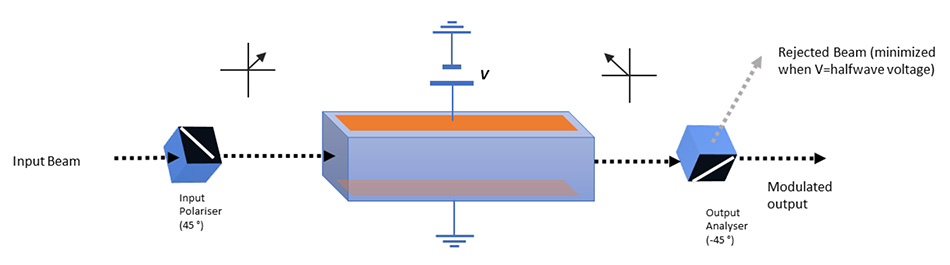
图 1: 横向普克尔斯盒操作的简化表示。 通过调整施加的电场调制透过分析仪的透射率。
普克尔斯盒由于设计比较简单,在双光子显微成像中得到了广泛应用,尤其适用于常见的激光波长,用户自己搭建的双光子系统。
例如,磷酸二氘钾 (KD*P) 普克尔盒可为 1100 nm 的 双光子成像 提供出色的透射率、速度和对比度特性,并提供适中的激光功率。 此外,KD*P 具有很低的群速度色散特性,从而显著减小群延迟色散 (GDD)。 对于没有色散预补偿以及波长调谐范围有限的超快激光器(例如钛宝石激光器),KD*P 普克尔斯盒是一种很好的选择。
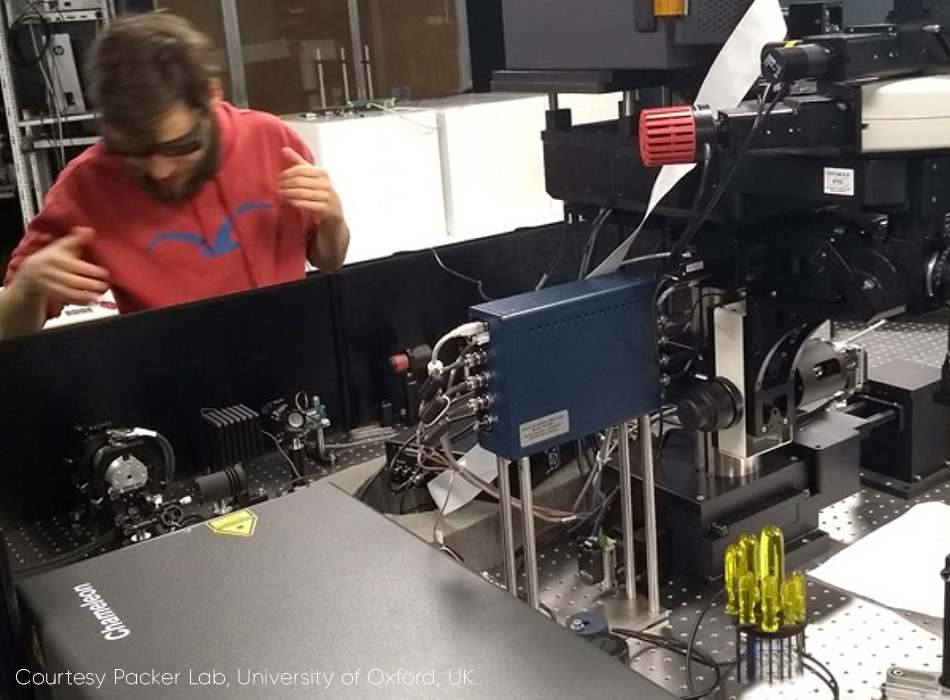
图 2: 双光子显微镜上的典型普克尔斯盒部署。 EOM 位于用户右下方。 英国牛津大学 Packer 实验室提供照片。
声光调制
声光调制器 (AOM) 包含一个透明晶体或玻璃,其上附有一个压电换能器。 施加到该换能器的射频 (RF) 波会诱发声波,使晶体产生应变,从而形成折射率光栅。 穿过细胞的光会经历布拉格衍射。
可实现的上升/下降时间与声波穿过激光束所需的时间成正比,因此可以通过减小晶体中的光束宽度进行优化。
辨别力和对比率由第零个和第一个衍射极之间的分离角 (θS) 以及到关注的工作平面的距离进行定义。
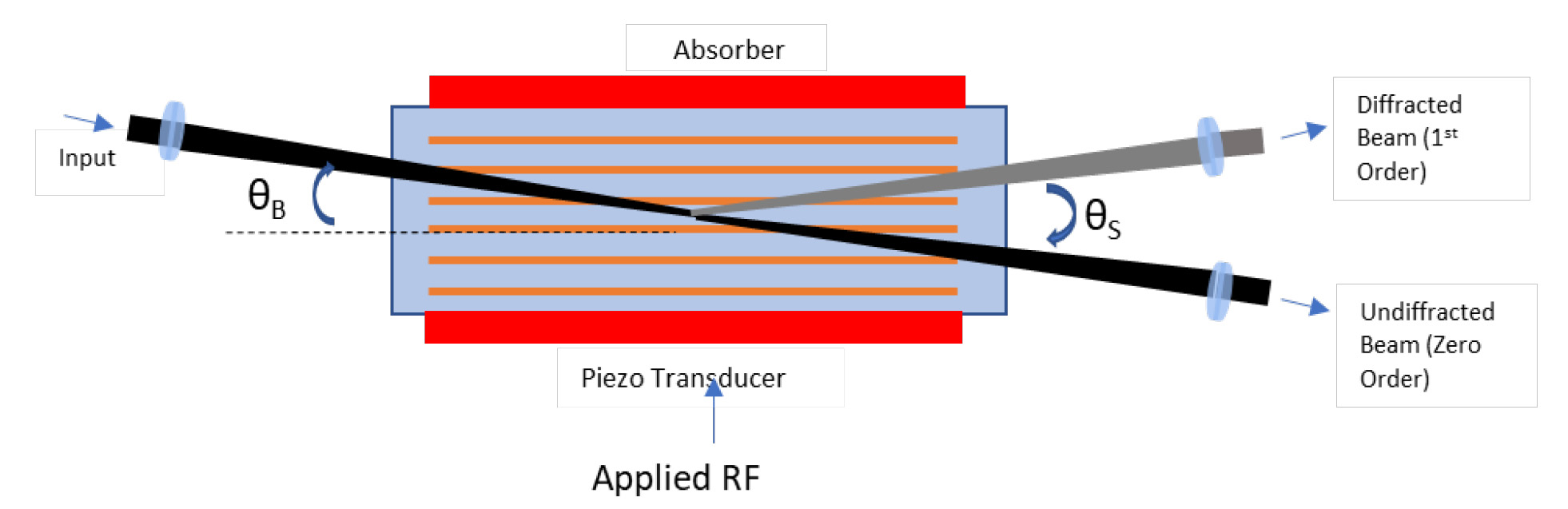
“约 680-1300 nm 波长且功率超过 2 W 的一体式宽可调谐激光器的出现,需要为激光调制的性能和集成工作实施一种新的机制。”
双光子显微成像中最常用的 AOM 材料是二氧化碲 (TeO2)。 这种材料在很宽的波长范围内具有出色的衍射效率和高功率处理能力。 使用 30 dBm 左右的适中 RF 功率时可实现高透射效率。
TeO2 AOM 通常配置在布拉格相互作用区中,该区提供优异的一阶衍射效率,并彻底湮灭高阶。 请注意,要以极低的 RF 功率级实现高效率,需要长度大于 1 cm 的晶体,从而导致不可忽略的群延迟色散 (GDD)。 另外考虑到其他下游光学器件的色散,尤其是物镜,基于 AOM 的显微镜系统可以通过与配备色散预补偿功能的激光器联合使用而受益,以在样品平面上维持尽可能短的脉冲。
为可调谐激光器部署 AOM 需要细致的光学和控制电子学设计。 由于分离角 (θS) 取决于 RF 驱动频率(即光栅周期)和激光波长,因此必须仔细校准 RF 驱动频率,以确保在调谐激光波长时显著减小指向变化。 此外,还应在不同 RF 功率下为不同波长实现高衍射效率。 尽管 AOM 具有出色的性能特点,但由于需要仔细控制 RF 频率和功率,并需要在可调谐成像系统中管理相对较大的 GVD,因此集成工作变得更加繁重,到目前为止,这已经限制了 AOM 在许多自制者和定制场景中的使用。
宽可调谐激光器的调制
约 680-1300 nm 波长且功率超过 2 W 的一体式宽可调谐激光器的出现,需要为激光调制的性能和集成工作实施一种新的机制。
通常使用的 KD*P 普克尔斯盒在高功率下会显示热晕效应,这对光束指向、束腰完整性和寿命都是有害的。 更长的波长会进一步带来更高驱动电压和对比率挑战。 钽酸锂是一种可行的 EOM 材料,可用于更宽的调谐,但商业装置的群延迟色散高于色散补偿激光器的可校正范围,从而导致更长的脉冲并降低峰值功率,不利于高效成像。
如前所述,尽管具有潜在的成本和性能优势,但基于 AOM 的解决方案需要高度的光学设计和电子控制专业知识才能部署,许多生物成像设施中通常并不具有此类装置。 即便如此,仍可作为一种集成解决方案从某些显微镜供应商购买 AOM 解决方案。
2017 年,相干公司意识到,将 AOM 调制与激光源集成在一起的配套式解决方案将会让用户和显微镜行业受益。 基于从工业超快加工激光器的集成 AOM 解决方案中收集到的专业知识,相干公司开发了全功率控制 (TPC),作为 Chameleon Discovery 激光器的完全集成选项。
自动免持包装中的 Chameleon Discovery NX 上提供的全功率控制可在 660 nm 至 1320 nm 的整个全倍频程调谐范围内提供高对比度 (>1000:1) 和高速(上升时间 <1 μs)调制。
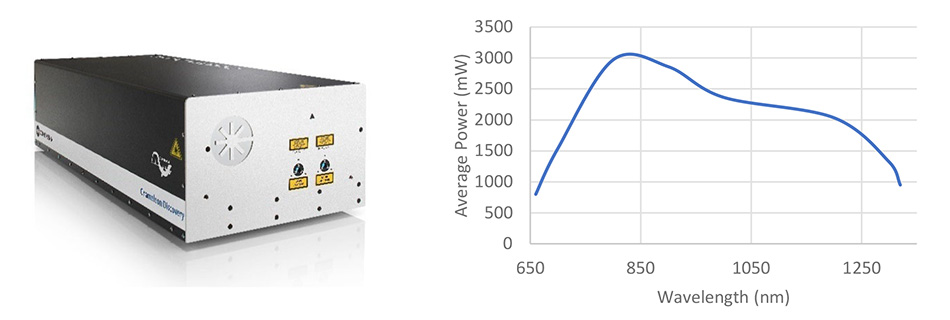
图 3: Chameleon Discovery NX TPC 和调制后的典型最大输出功率。
RF 频率和功率校准与调整的所有繁琐要求都已在激光器内部进行编程,因此用户或显微镜集成商只需提供所需的设定波长和功率级即可。
由于 AOM 的成本效益很高,因此 Chameleon Discovery NX TPC 的固定波长 1040 nm 输出还配备了自有的专用 AOM 和驱动器。
可以通过串行/USB 命令或快速模拟控制输入,方便地进行控制。
图 4: 可以使用提供的 GUI 直接更改输出功率,
或者用户可以为逆程消隐和快速抖动控制提供
额外的快速模拟输入。
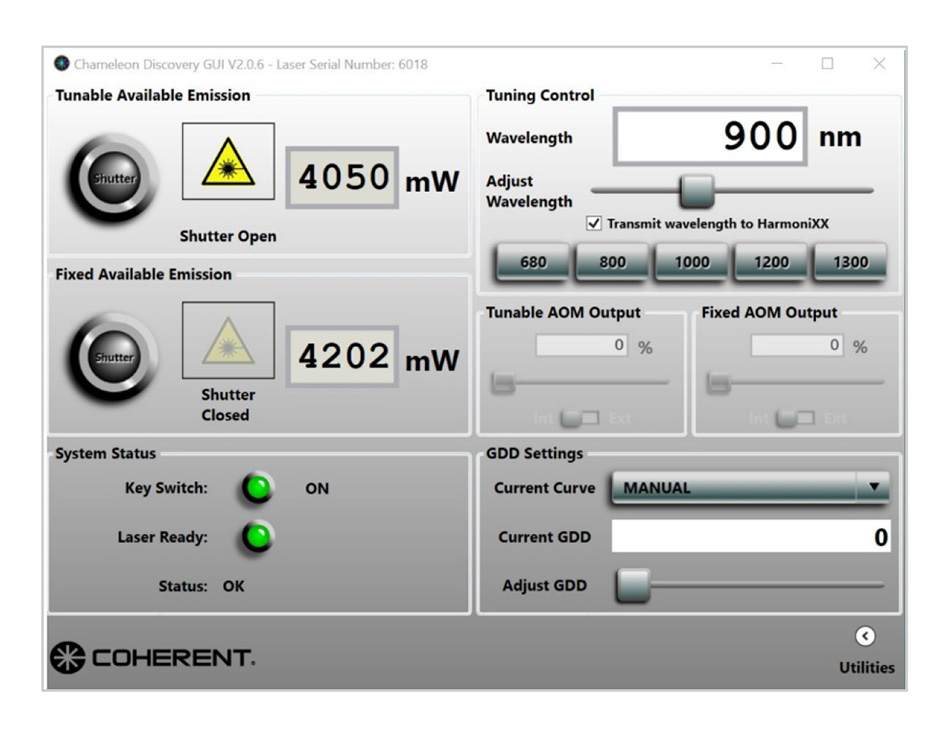
未来趋势
随着双光子成像技术应用范围向 OEM 和临床前应用的进一步拓展,人们对经济高效的单波长飞秒光源的需求日益增长。 Axon 系列紧凑型超快光源完全满足了这些要求。
从产品概念设计阶段开始,TPC 功能就被集成到了 Axon 设计中,以简化向新显微镜设计和应用中的部署。 对于双光子显微镜系统只是活动式诊断、临床或高通量药物筛选设备的一部分而不是单纯研究仪器的应用,这带来了终极集成便利性。
在尖端神经科学研究中,高功率激光器在使用光遗传学刺激的全光体内成像技术中发挥着关键作用(Yuste,2012 年)。 数十瓦的激光功率通过空间光调制器 (SLM) 拆分成能够单独处理数十或数百个神经元的单独细光束。 这种光控制方法需要短且可定制的突发脉冲。 由于采用全光纤设计方式,相干公司 Monaco 这样的高功率光纤激光器可提供此类应用所需的灵活性。 由此产生的高平均功率、高能量激光要求以及在亚毫秒时间尺度上切换刺激光束的需求为现有的普克尔斯盒技术带来了具体挑战。 为此,AOM 技术已完全集成到 Monaco 中,以实现精致的脉冲控制、简化的显微镜设计并提高成像系统的可靠性。
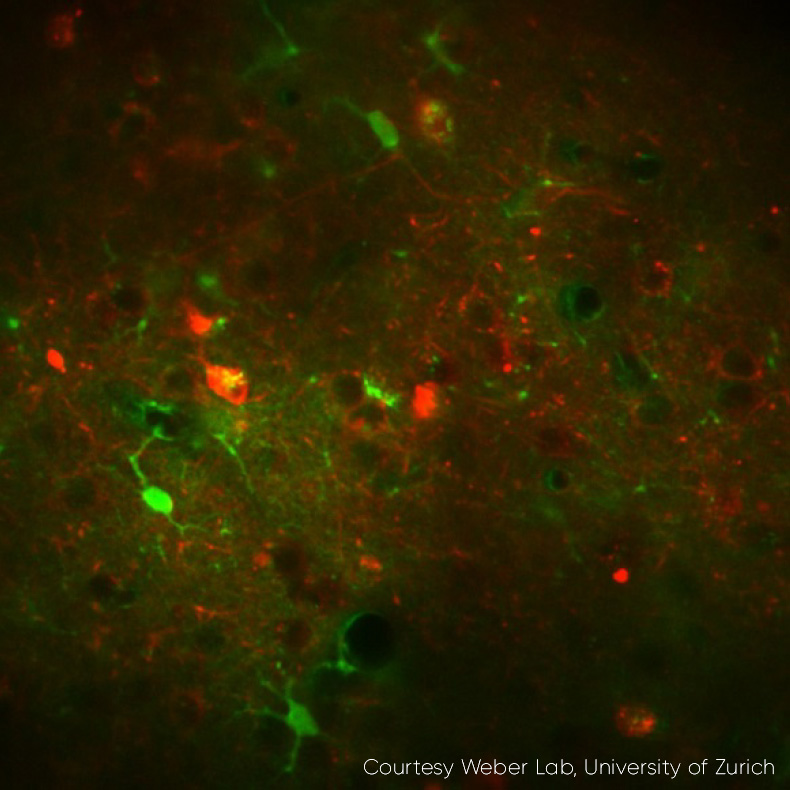
图 5: Discovery TPC 支持的高对比度、快速帧速率钙成像示例。 (在 1100nm 激光激发下表达 RCaMP1.07 的神经元(红色)和在 940nm 激光激发下表达 GCaMP6s 的星形胶质细胞(绿色)的重叠图,小鼠活体内。 激发源 Chameleon Discovery TPC。 苏黎世大学 Weber 实验室供图)。
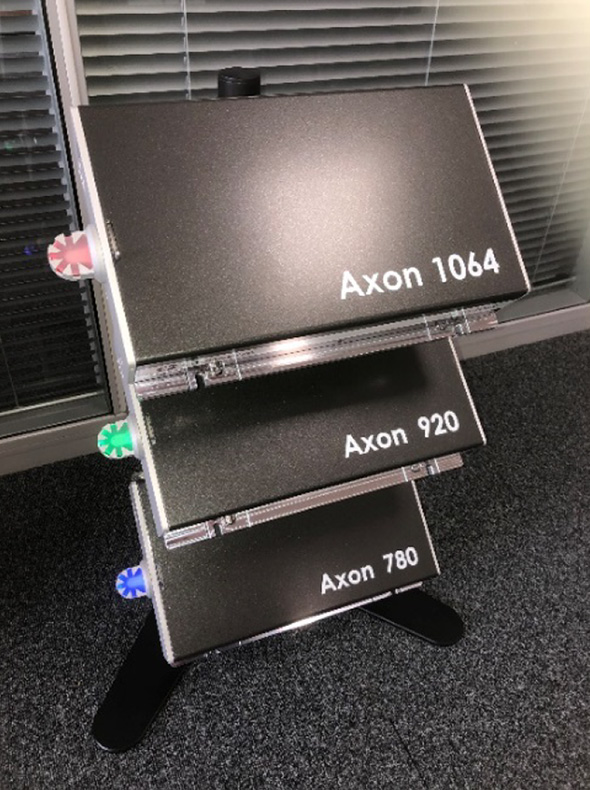
图 6: 所有 Axon 激光器都以共同的外形尺寸提供 TPC 功能选件。
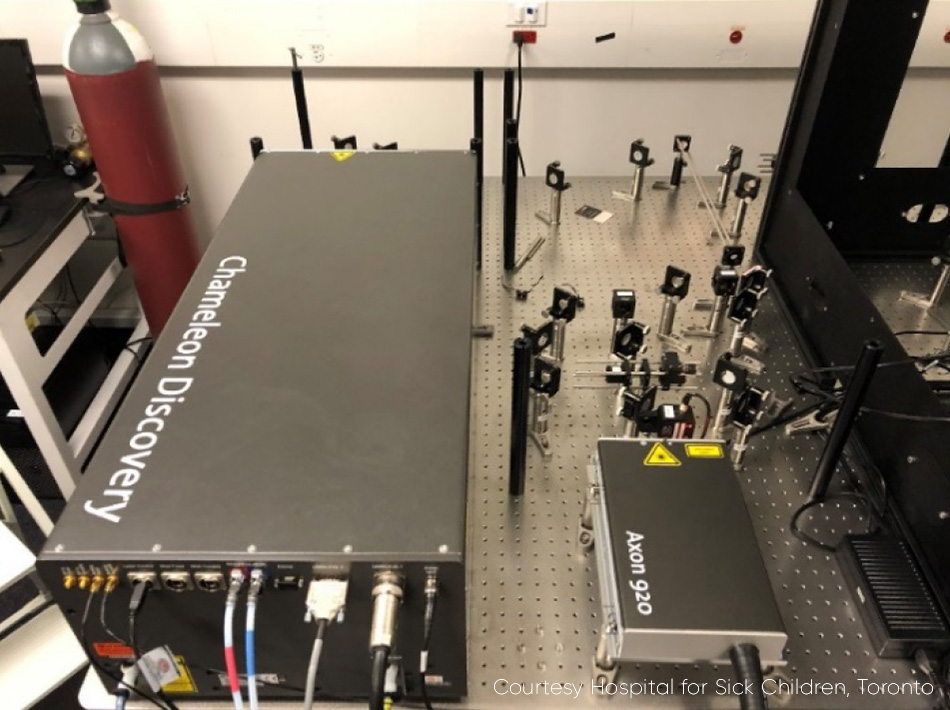
图 7: Chameleon Discovery NX TPC 与 Axon 920 TPC 联合使用。 TPC 可简化光学布局并节省宝贵的载台空间。 照片由多伦多儿童医院 Neil Merovitch 提供。
总结
在本技术说明中,我们讨论了对双光子显微成像中使用的飞秒激光器的激光输出功率进行调制的两种主要方法:电光调制和声光调制。 迄今为止,大多数“自制者”都选择了 EOM,因为在光路中部署这种高压驱动设备相对简单。 显微镜的供应商们将其 EOM 或 AOM 部分集成在其激光传输系统中,利用其软件架构同时控制显微镜和激光。 相干公司利用其为制造 24/7 环境设计的高功率光纤激光器的制造经验,意识到 AOM 方法在尺寸、成本、速度和整体性能方面的优势也将满足双光子成像应用的要求。 无论是先进的神经科学应用还是医学诊断应用,通过将 AOM 的复杂控制集成到 Discovery NX、Axon 和 Monaco 的激光软件和硬件架构中,双光子用户(自制者和显微镜公司)可以从大大简化且易于控制的光学装置中受益。