Lasermikroskope sind wichtige Werkzeuge in der Biologie
Die heutigen konfokalen Mikroskope, Multiphotonenmikroskope, Super-Resolution-Techniken wie PALM und STORM, Lichtblatttechniken und vieles mehr sind alle auf Laser angewiesen, um die Grundlagen des Lebens auf zellulärer Ebene zu entschlüsseln
19. Oktober 2021 von Coherent
The use of the optical microscope in life sciences dates back to the time of Anton van Leeuwenhoek, a Dutch businessman, who famously used it to get the first glimpses of tiny life forms. Fast forward about 400 years and scientists across the life sciences still rely on optical microscopes as critical tools in their work. That’s pretty impressive longevity by any measure. And the word “microscopic” of course has long been a universally accepted term used to refer to anything small.
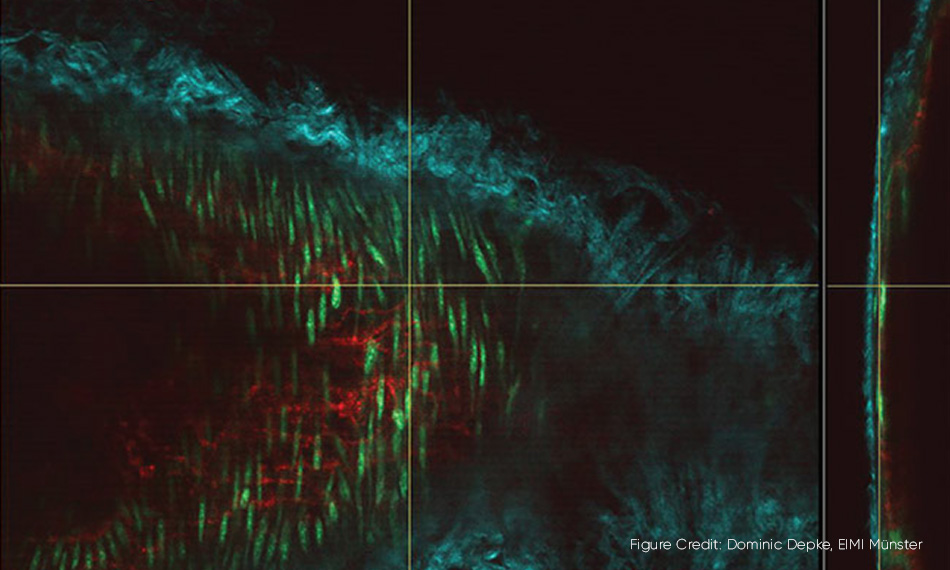
3D microscopy in action. A mouse carotid artery where several cell components are visualized as different colors using two wavelengths from a Chameleon Discovery laser. The main image shows the carotid artery “en face” and the vertical slice on the right is an orthogonal view of the artery wall.
Mapping life with microscopes
Why is the optical microscope still so important and what does that have to do with lasers? Well, it’s the simplest instrument to image the structure of small things. But just as important, it’s evolved to become the only instrument that can tell you what those structures are made of. By manipulating and measuring the colors (wavelengths) of light that interact with a sample, scientists can produce a map of all kinds of different biochemicals. And since every living organism, from a single cell amoeba, to a tree, to an elephant, are all based on complex biochemical reactions, that’s a pretty useful capability.
This chemical mapping used to be rather crude. A hundred years ago, a dead (“fixed”) sample of a plant or an animal would be chemically treated with a colored dye called a stain. This might attach to all the fat in the sample, or maybe all the protein. The viewer could then see what bits of the sample were colored by this dye and which bits were not.
Today’s scientists have myriad dyes to choose from and their studies are far, far more sophisticated. Most of these are fluorescent chemicals often called fluorophores or fluorochromes. (Fluorescent materials absorb light at one wavelength and re-emit the light at a different, longer wavelength.) Some fluorophores are just chemicals that come right out of a bottle, but often they are fluorescent proteins that the plant or animal has been genetically modified to produce directly itself.
A laser is the ultimate microscope light source
But what about lasers? Well we’re coming to that now. It turns out that the laser is the ultimate light source for doing fluorescence microscopy for several reasons. Scientists want to use microscopes to see ever higher detail. We call that spatial resolution, or just resolution. They also want to look at real 3-dimensional things, not just thin dead slices. And they also want to use microscopes to observe live biology happening in real-time. Lasers have turned out to be the light source that unlocks all of that.
Lasers bring several killer advantages to fluorescence microscopy. First, a laser emits light at only one wavelength. And thanks in part to our optically pumped semiconductor (OPSL) technology, this wavelength can be chosen to match the absorption of specific fluorophores. Glass filters in front of the microscope’s camera then block the laser light scattered by the sample (i.e., glare) and make sure only the fluorescence is selectively imaged. Now you could get a single wavelength band using a lamp or a LED with a filter. But the laser output beam can be focused to a much smaller spot than light from any lamp or LED. This is key to the confocal laser scanning microscope (CLSM) which delivers 3D images without all the out-of-focus background fluorescence that would otherwise make that impossible.
Lasers can also deliver a much higher intensity than a lamp or LED; so even faint fluorescence can be imaged fast. Plus the laser’s high and adjustable intensity are key to enabling the latest super-resolution techniques (e.g., PALM, STORM) that produce images with resolution down to a few nanometers. And that’s a big deal that won a Nobel Prize in 2014, because as recently as 40 years ago, it was thought you could never take a visible microscope past the hard limit of diffraction, i.e., about 250 nanometers.
Taking it live
In many areas of life sciences, most notably neuroscience, researchers want imaging depth just as much as resolution. They want to get sharp images deep inside tissue or even inside whole organisms. And they want to do this on live tissue. While confocal and super-resolution methods are great for fixed samples, they typically cause too much photodamage for live specimens. Fortunately, a technique called multiphoton microscopy meets this challenge enabled by ultrafast lasers. (Yet another Nobel prize!). In fact, this is such an important application for ultrafast lasers that Coherent makes a whole family of Chameleon ultrafast lasers, tailored just for multiphoton microscopy.
Looking to the future, scientists are developing tools for medical applications such as real-time biopsies, based on multiphoton microscopy. That’s possible because some of the multiphoton methods don’t even need a dye or fluorescent protein at all! These are collectively called label-free imaging.
So that’s just a microscopic glimpse into the massive impact of the laser microscope in biology. We’d like to think that van Leeuwenhoek would be mightily amazed and impressed, because we are!