Faraday Rotators and Isolators
What are Faraday Rotators and Faraday Isolators?
A Faraday rotator is an optic that rotates the polarization orientation of light. It consists of magneto-optic crystal placed in a magnetic field. A Faraday rotator is often combined with other polarization components to make a Faraday isolator, which is essentially a one-way valve for light.
Faraday rotators and Faraday isolators provide a unique set of capabilities and characteristics for manipulating polarized light, especially as compared to waveplates or other birefringent optics (the other widely used components for polarization control). As a result, they have found use in applications as varied as industrial and medical laser systems, optical signal processing, optical sensing, telecommunications, and scientific research.
The most important of these unique properties is that Faraday rotators always rotate polarization in the same direction – no matter which way light enters the device. So, if the rotator is configured to rotate polarization by 45° clockwise for light passing through it in one direction, it will again rotate it 45° in the same direction for light traveling in the opposite direction. The two trips back and forth will produce a total polarization rotation of 90°.
This isn’t the case for the half waveplate. If a waveplate is configured to rotate the polarization of light by 45° in one direction, it will rotate it back that same amount when light goes through it the opposite way. The total double pass rotation will be 0°.
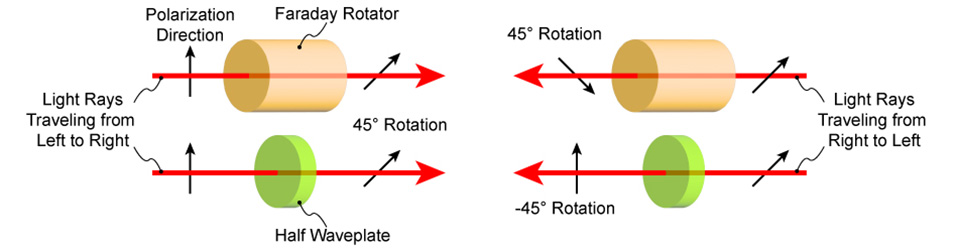
A Faraday rotator always rotates polarization in the same direction. So, if it is set to rotate light 45°, two passes through the device back and forth will produce a total rotation of 90°. In contrast, a half waveplate configured to rotate input polarized light 45° will simply rotate returned light back to its original orientation – a net rotation of 0°.
Another important distinction is that the rotation angle produced by a Faraday rotator is determined by the applied magnetic field. If an electromagnet (rather than a permanent magnet) is the source of this field, then the amount of rotation can be controlled electronically. In contrast, a half waveplate must be physically rotated to change the amount of rotation it produces.
What are Polarization and the Faraday Effect?
To better understand what a Faraday rotator does, it’s first necessary to take a step back and talk briefly about polarization. And to understand polarization we need to talk about the wave nature of light.
Light is an electromagnetic wave. Of course, we’re all familiar with water waves. Imagine a stone thrown into a pond. The ripples that spread out across the water are waves. That is, they’re periodic variations in the surface height of the water that travel out from the center.
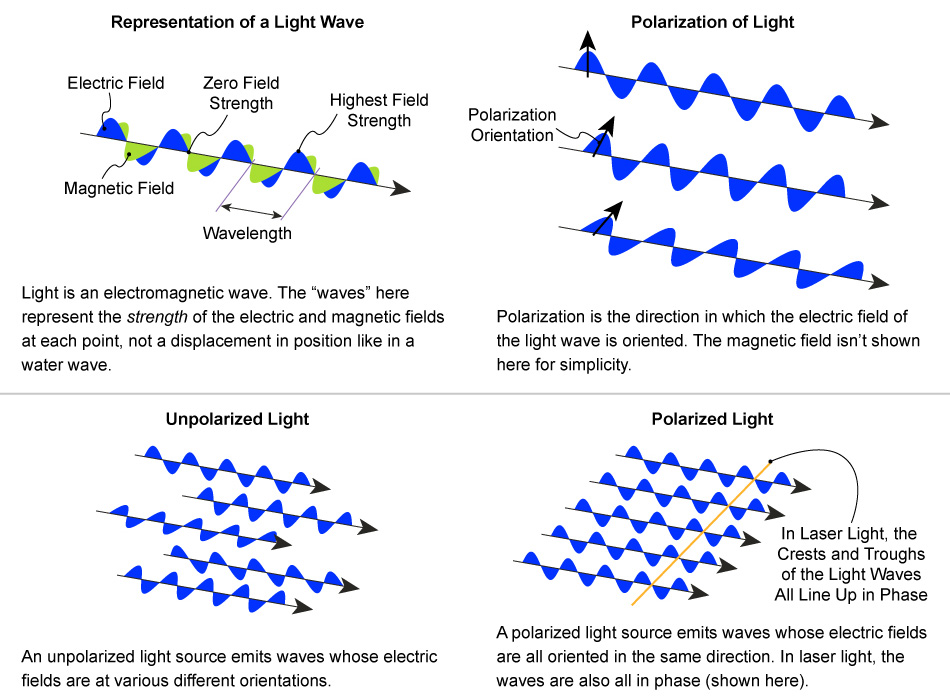
Simplified representation of light as an electromagnetic wave, and the concept of polarization.
Now imagine that instead of waves that are ripples in water surface height, we have waves consisting of electric and magnetic fields. This means the strength of these fields changes periodically over distance just the way the surface height changes in a water wave.
Polarization is simply the direction in space in which each light wave’s electric field is oriented. Because, keep in mind, unlike a water wave which is confined to the surface of the pond (meaning the water height can only vary up and down), light waves can travel in any orientation and direction. They don’t need any medium in which to propagate.
What physicist Michael Faraday discovered in 1845 is that when some materials (called magneto-optic materials) are placed in a magnetic field, the polarization direction of light waves traveling through them will rotate. The amount of this rotation is directly proportional to the strength of the magnetic field, the distance the light travels inside the material, and the material’s Verdet constant. The Verdet constant is simply a measure of the strength of the magneto-optic effect in that specific material. It is usually determined by measurement.
While most transparent dielectric materials are magneto-optic, the effect is usually very weak. However, there are a few materials that have a large Verdet constant. Typically, these are glasses or crystals containing the element Terbium. In particular, the crystal terbium gallium garnet (TGG) exhibits a strong magneto-optic effect and has low absorption at commonly used wavelengths. It also has various other desirable physical properties and is relatively low cost. That’s why TGG is one of the most commonly used materials for making Faraday rotators and Isolators.
What is a Faraday Isolator?
There are a variety of photonic components that can be constructed based on the Faraday rotator, and the Faraday isolator is one of the most useful and widely employed of these. It allows polarized light to pass through unimpeded in one direction but attenuates most light that enters from the opposite direction.
A common use of the Faraday isolator is at the output end of a laser or laser amplifier to protect it from back reflected light. Specifically, this is light reflected back towards the laser by other optics in the system, or by the object the laser is illuminating (for example, a reflective piece of metal being welded by an industrial laser). If powerful enough, back reflected light can damage a laser. But even at much lower levels, back reflected light can cause laser operating instability, like noise and power fluctuations.
The operation of Faraday isolators is conceptually simple and is illustrated in the drawing. Linearly polarized light (coming in from the left) passes through a polarizer (#1) aligned with its polarization. It enters a Faraday rotator which rotates the polarization by 45°. The light passes through another polarizer (#2) aligned with this rotated polarization and then into the optical system and process. This configuration allows virtually all the laser light to pass through the device unattenuated.
Any light returned from the optical system or process first passes through a polarizer (#2) which rejects all polarizations different from the original isolator output. This filtered light then passes through the rotator and undergoes another 45° rotation. This puts its polarization at right angles to its original orientation. This means that it will be rejected by the first polarizing beamsplitter (#1).
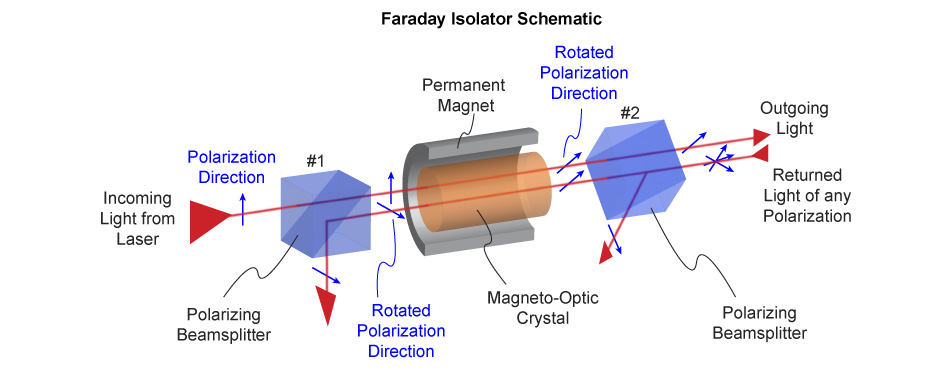
The basic operating principles of a Faraday isolator.
Designing and manufacturing practical Faraday isolators requires balancing several factors. Key parameters are typically aperture size, wavelength range, transmission (attenuation in the forward direction), and isolation (blocking of returned light). Overall maximum laser power rating and laser-induced damage threshold (LIDT) are also often considerations. And, of course, these are all traded off against cost, and sometimes physical size or weight.
Optimizing for these various parameters requires making design choices and tradeoffs in the strength and size of the permanent magnet, the required quality of the magneto-optic material (specifically in terms of absorption, index homogeneity, and birefringence), the types of thin film coatings used, and more.
As a result, Faraday isolator manufacturers like Coherent offer a range of different products, each optimized for different tasks. Examples include our compact Low Power Rotators and Isolators for near-IR seed lasers, EURYS Rotators and Isolators for Ti:Sapphire oscillators, and TORNOS Rotators and Isolators specifically designed to prevent optical feedback in lasers from 405 nm to 980 nm.
Novel Technology for High-Power Isolators
TGG has long been the Faraday rotator crystal of choice for the 650 – 1100 nm spectral range for several reasons. For example, it can be grown with high purity. It has a high Verdet constant and its symmetric cubic crystal structure and low intrinsic birefringence make it easy to achieve high isolation without the need for sensitive alignment processes. And it is relatively low cost.
However, even the purest TGG eventually encounters performance limits due to its bulk absorption. This absorption causes localized heating within the crystal with performance-limiting consequences. As the output power of industrial lasers has continued to scale up over the past decades, TGG’s inherent absorption and thermo-optical properties have become increasingly disadvantageous.
Potassium Terbium Fluoride (KTF) is another magneto-optic material with a similar transmission range to TGG, as well as a comparable Verdet constant. Most importantly, it possesses a lower bulk absorption coefficient (eight times lower), thermo-optic coefficient (15 times lower), and stress-optic coefficient than TGG. Together, these allow it to avoid the degradations in isolation performance, beam focus, and beam quality that plague TGG-based Faraday isolators when exposed to very high laser power.
Early KTF growth efforts produced boules with bubbles, inclusions, and issues of high scatter. These provided no net improvement in transmission over TGG. But Coherent has pioneered numerous process refinements that now deliver improved yields of high-quality KTF at a reduced cost. This has enabled us to produce a cost-competitive series of Faraday isolators intended specifically for high-power lasers – the Coherent Pavos Ultra series – that incorporate this material.
Learn more about Coherent isolators and rotators, and kW-class Faraday isolators.