Cancer Cell Analysis — A New Vector
A combination of a laser innovation and clever instrument innovation is providing a new high-throughput route to potentially important diagnostic data about breast cancer cells.
July 31, 2023 by Coherent
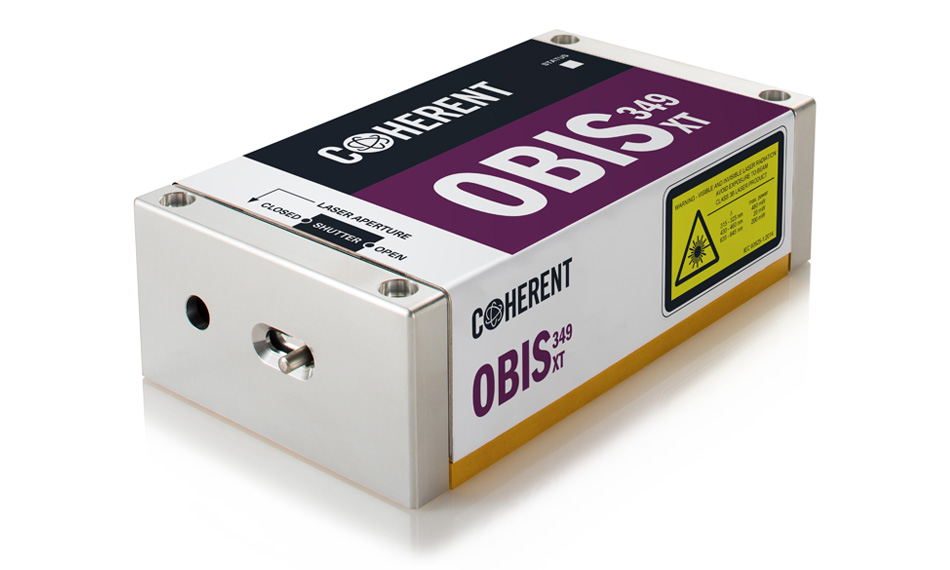
What is flow cytometry?
In flow cytometry, cells or other bio-particles are forced to flow in single-file and at high-speed through an interaction zone where they intersect with one or more focused laser beams. The resultant laser-excited fluorescence intensity, and often the scattered laser light intensity, is recorded for every cell. In counting instruments, this allows large populations of cells to be quickly analyzed, e.g., to see what percentage of the cells are of a particular type, or types. In instruments configured to also support sorting, cells of a targeted type are separately collected by deflecting them into a receptacle using an electric field, again based on the fluorescence/scatter signature of each cell.
Flow cytometry is fast, making it suitable for many different applications. It is used extensively in medicine for blood cell analysis, e.g., the complete blood count or “CBC.” It is also used in research labs and in drug discovery, often to analyze other types of mammalian cells beyond blood cells. It is sometimes used to study tiny organisms in ocean water and to sex-sort sperm for animal husbandry, e.g., to favor the production of female offspring in dairy cattle.
Using more wavelengths and ultraviolet wavelengths
Even though it has been around for decades, flow cytometry is quite a dynamic field. A big trend is called “multiparameter flow cytometry” where as many as 12 different laser wavelengths are used to analyze cells for a large number of different parameters. Laser manufacturers support this trend by offering lasers at new wavelengths all in a common format, as well as by packaging multiple lasers in a turnkey light engine. (The OBIS product line from Coherent embodies both of these developments.)
Another trend is the increasing use of ultraviolet (UV) laser wavelengths. This allows expansion of the multi-parameter concept and it also enables the use of autofluorescence. This refers to materials found in cells that naturally fluoresce without the use of fluorescent dyes or other additives. These materials include some important metabolites, NAD(P)H and FAD.
Researchers using the time to figure things out
In a previous Coherent Success Story, we described how the research group of Professor Jessica Houston (New Mexico State University) are pioneers in the development and use of time-resolved flow cytometry. We discussed why they are targeting NAD(P)Hand FAD; this allows them to not only count cells, but also determine their metabolic condition. Dr. Houston explains, “Most flow cytometry measurements are based on the intensity of the fluorescence signals. But there are a lot of factors that can introduce noise and errors to this measurement, including how much fluorochrome is bound to the surface or interior of the cells. We decided to also look at the decay times of the fluorescence signal from each cell. The lifetime is independent of the concentration and it depends on the chemical state of the fluorochrome or natural (endogenous) material responsible for autofluorescence. Endogenous fluorescence can give us unique information about the metabolic condition of a cell. For example, we can use the information to assess whether a cell is functioning normally or is a transformed (cancerous) cell.”
They are particularly interested in NAD(P)H and FAD because the fluorescence lifetime of these cofactors indicates whether they are bound to proteins or not. In turn this indicates how the cell is oxidizing sugar to get energy, primarily by processes called oxidative phosphorylation and glycolysis. In many cancer cells, and otherwise “faulty” or distressed cells, reports show high levels of measured “free”, unbound NAD(P)H, which indicates cells are making energy through the less efficient process called glycolysis. The ratio of the amount of FAD relative to NAD(P)H measured optically indicates the metabolic activity in the cell and is called the Redox Ratio.
A clever trick with CW lasers
At first, the Houston group used pulsing of the laser beam to get time-dependent data. The instrument then records the decay of the fluorescence pulse in one of two ways. Either as real-time decay curves as the laser excitation is switched off, or in the frequency domain as the laser is repeatedly modulated at very high speed. This latter method is how the group used an OBIS laser at a wavelength of 375 nm to monitor FAD. But to accurately determine the complete Redox Ratio ideally requires measuring both FAD and NAD(P)H. And to independently measure NAD(P)H fluorescent decay times requires excitation even further in the UV – at around 349 nm.
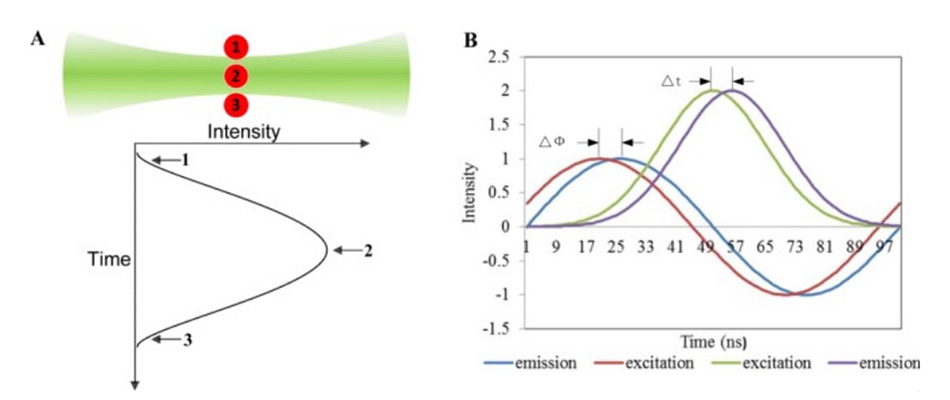
Recently, Coherent supplied Houston’s group with a new OBIS model, developed to output 349 nm. However, this uses different internal technology to the 375 nm lasers and is not as simple to directly modulate. This was no problem for them because a few years ago, the group developed a clever technique called non-modulated fluorescence lifetime cytometry (nFLIC) that uses a continuous wave (CW) laser to determine the average fluorescence lifetime.
Figure 1 shows how this works. By using a laser with a perfect TEM00 output beam, when a cell transits through the focus, the excitation probability rises and falls across the Gaussian beam profile. But the non-zero fluorescence decay time causes something called the fluorescence pulse-delay (FPD). As the cell passes through the beam, the fluorescence intensity falls away more slowly than the Gaussian excitation profile. And the Houston team rigorously proved that the FPD is a valid representation of the average fluorescence lifetime of the cell.
The new OBIS 375 has worked very well for nFLIC studies in the Houston lab because of its superior output beam qualities. Professor Houston explains, “First, it’s got the nice clean TEM00 beam shape we need. It’s also a very low-noise laser. And just as important, the power, beam shape, and beam pointing are very stable. This matters because it enables robust calibration of the relationship between fluorescence pulse-decay and fluorescent lifetime using special fluorescent beads."
Cancer cell resistance
Cancer cells are a formidable foe because they often develop resistance to otherwise powerful chemotherapy drugs. At the CYTO 2023 Conference (Montreal, Canada), Samantha Valentino – a graduate student in the Houston lab – gave a standout presentation showing the value of using time-resolved flow cytometry to determine FAD/NADH fluorescence lifetimes and redox ratios as a powerful tool to monitor breast cancer resistance to a chemotherapy drug called Tamoxifen.
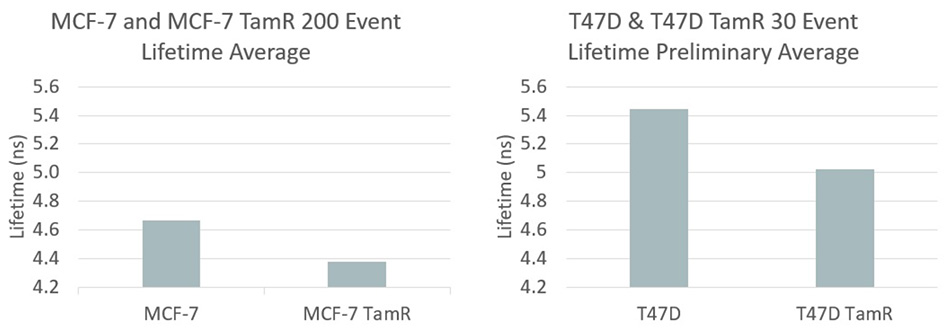
Figure 2. Fluorescent lifetime data for MCF and T47D breast cancer cells – a comparison between sensitive and resistant cell lines. Graphic courtesy Houston lab.
Specifically, she explained that Tamoxifen is a widely used drug that is effective against breast cancers characterized as “estrogen receptor positive.” It is used to achieve remission. However, resistance typically develops within 2- 5 years of beginning treatment. Valentino began by noting that the redox ratio is often more skewed in more aggressive cancer cells, such as drug-resistant cancers. She studied two different breast cancer types (MCF-7 and T47D). In both cases, she compared cancer cell lines that were Tamoxifen sensitive with cell lines that were Tamoxifen resistant. Figure 2 summarizes the results, showing that their approach revealed a statistically significant difference in the sensitive and resistant cell types. It goes without saying that the ability to rapidly analyze cancer cell populations for their drug-resistance characteristics could turn out to be an important new tool in the cancer-fighting arsenal: both in drug discovery and research, and to provide improved patient-specific treatments.
Summary
Analytical techniques based on laser-excited fluorescence like flow cytometry, DNA sequencing, and microscopic tissue biopsies are increasingly vital tools supporting the trend of personalized medicine, which was further accelerated by the recent Covid-19 pandemic. Coherent is strongly committed to supporting advances in these techniques with laser tools that improve their speed and data quality, lower the barrier to use, and decrease the overall instrument cost.